Introduction
Thermogravimetry has the potential to provide valuable information for the characterization of organoplatinum antitumor agents. For well-behaved systems, characteristic mass losses may suggest the identity of ligands present and the residue from thermal degradation will reflect the platinum content of the compound [1,2].
Several developments have impacted the formulation of effective, non-toxic, sustained action organoplatinum antitumor drugs. The first was the serendipitous discovery of the remarkable biological activity of cis-dichlorodiammineplatinum(II) (Cisplatin) [3-10]. Cisplatin is a broad-spectrum cancer drug effective against a wide range of tumors. For many years Cisplatin was the most widely used anticancer drug. It is often used in combination with organic antitumor compounds or with Carboplatin [diammino(1,1- cyclobutanedicarboxylato)platinum(II)], the second platinum anticancer drug to gain widespread commercial use. Carboplatin displays a somewhat different toxicity profile than does Cisplatin, making it an attractive compliment to Cisplatin [11-13]. All the compounds shown below reflect the structure required for antitumor activity (two inert cis ligands and two labile ligands; chlorine displays a near optimum hydrolysis rate under physiological conditions: halflife of about one hour at 37 oC).
The potential of these drugs has been limited because of severe side effects which accompany their administration. Among the most debilitating side effects induced by organoplatinum drugs are severe kidney damage [14] and extreme nausea (as a class, the platinum compounds are among the most effective nausea producing agents known, to the point that some patients refuse to complete the treatment regimen) [15,16]. In an attempt to identify active but less toxic drugs literally hundreds of platinum compounds in which the structure of the amine ligand has been varied have been synthesized and evaluated for antitumor activity. In the main, this has been a fruitless undertaking. While some ligands impart better solubility, activity, or toxicity than similar properties associated with compounds derived from simple ammonia ligands, no compounds with clearly superior performance have been found. Of the hundreds of compounds synthesized, fewer than thirty have entered clinical trials as antitumor agents [17,18].
A second development has been the huge progress in the utilization of polymeric carriers for a variety of drugs [19-27]. The use of a polymer-drug conjugate may provide a number of advantages over the drug alone. These may include increased water solubility, sustained delivery of drug, reduced toxicity, enhanced biodistribution, and preferential penetration of abnormal tissue.
A third development has been the discovery of dendritic polymers [28,29]. Dendrimers offer multiple modes of drug incorporation. In particular, the poly(amidoamine) [PAMAM] dendrimers are attractive as drug carriers. They are water-soluble, multivalent, and in general, nontoxic [30-32]. Dendrimers are highly branched macromolecules with precisely controlled size, shape and end-group functionality. They represent unique core-shell structures consisting of three basic architectural components: a core, an interior of cells (generations) that have repeating branchcell units, and terminal functional groups (outer shell or periphery). Each generation is built sequentially from a predecessor to form a symmetrical, nearly monodisperse structure of precise molecular weight and nanoscale dimensions [33]. For PAMAM dendrimers halfgeneration structures are carboxyl-terminated; full generations are amine-terminated. Both types of functionality are useful for drug conjugation [34,35]. Alternatively, simpler hyperbranched polymers may be used in a similar manner for drug conjugation [36].
A fourth development has been the discovery that functionalized, water-soluble carbon nanotubes are able to traverse the cell membrane by endocytosis to deliver a chemotherapeutic agent [37-42]. This has been exploited for the delivery of organoplatinum prodrugs [41,42]. Approximately 65 platinum (IV) units per nanotube may be loaded [42]. This is comparable to the number of (diamminocyclohexane) platinum (II) units that may be attached to the surface of a generation 4.5 poly(amidoamine) [PAMAM] dendrimer [34, 35]. Further, the multi-valent prodrug may be functionalized with a folate component to specifically target folate receptor-enriched tumor cells [41]. The prodrug may also be attached to a gold nanoparticle as a targeting agent [43].
Finally, a fifth development has been the recognition that platinum (IV) compounds could act as prodrugs for delivery of effective antitumor agents [41, 44-46]. With respect to their platinum (II) counterparts, platinum (IV) compounds possess a greater kinetic inertness. Thus, more of the platinum species may reach the targeted region prior to decomposition. When platinum (II) compounds are oxidized to platinum (IV) species two additional coordination sites become available for the introduction of functionality to enhance uptake via drug targeting, to inhibit resistance, to improve biodistribution, to modify lipophilicity, or to provide monomers for the generation of polymeric prodrugs. It is thought that platinum (IV) compounds are reduced to platinum (II) species in the cell which act as the effective antitumor agent for interaction with DNA (Scheme 1).
EXPERIMENTAL
General
Thermogravimetry was accomplished at a heating rate of 10 oC/ min. Samples (5-10 mg) were contained in a platinum pan.
The sample compartment was purged with dry nitrogen at 50 mL/min during analysis. Nuclear magnetic resonance (NMR) spectra were obtained using 10% to 25% solutions in dimethyl sulfoxide-d6. Proton and carbon chemical shifts were reported in parts-per-million (δ) with respect to tetramethylsilane (TMS) as internal reference (δ = 0.00). Infrared (IR) spectra were obtained using solid solutions (1%) in anhydrous potassium bromide (as discs). Absorptions were recorded in wave numbers (cm-1).
Materials
Tetrahydrofuran (THF) was distilled from lithium aluminium hydride in a nitrogen atmosphere prior to use; methylene chloride from calcium hydride. Potassium tetrachloroplatinate(II), cisdichloro( diammine)platinum(II), and nitrogen dioxide were used as received from commercial sources.
Synthesis
cis-Dichloro-trans-dihydroxy-cis-diammineplatinum(IV) [47,48]. cis-Dichloro-diammine-platinum(II) (3.01g, 0.01 mol) was suspended in 75 ml of water and 114 ml of 30% aqueous hydrogen peroxide (0.10 mol; 10 fold excess). The mixture was stirred at 50°C in a nitrogen atmosphere and the absence of light for an hour at solvent reflux. The mixture was allowed to cool and to stand at 5°C overnight for crystallization of the product. The bright yellow solid which formed was collected by filtration at reduced pressure and washed, successively, with cold water, ethanol, and diethyl ether. The solid was dried over anhydrous sodium sulfate at reduced pressure (15 torr) to afford 2.57 g (77.3% yield) of the dihydroxy compound.
cis-Dichloro-trans-disuccinato-cis-diamineplatinum(IV). A solution of 0.27g (2.67 mmol) of succinic anhydride and 0.21g (0.67 mmol) of cis-dichloro-trans-dihydroxy-cis-diammineplatinum(IV) in 5.0 ml of dimethylsulfoxide was stirred in nitrogen in the absence of light at 70°C for 24 hour. The solution was allowed to cool and the solvent was removed by lyophilization. The residual solid was suspended in dichloromethane to remove succinic anhydride. The insoluble material was collected by filtration at reduced pressure and washed with several portions of dichloromethane. Recrystallization from acetone (-23°C) afforded the disuccinato compound as yellow needles which were collected by filtration at reduced pressure, washed with cold acetone, and dried over anhydrous sodium sulfate at reduced pressure to provide 0.202 g (63.1 % yield) of the expected product [41,46].
cis-Dichloro-trans-hydroxo/ethoxo-cis-diamineplatinum(IV). To a stirred suspension of 0.50g (1.67 mmol) of cis-dichlorodiammine platinum(II) in 625 ml of ethanol maintained at 70°C was added 2.1 ml of aqueous hydrogen peroxide solution. The resulting mixture was stirred for 5 hours at 70°C. The mixture was allowed to cool and most of the solvent was removed by rotary evaporation at reduced pressure. The residue was treated with 75 ml of diethyl ether to provide a suspension of a bright yellow solid. The solid was collected by filtration at reduced pressure, washed, successively, with cold ethanol and diethyl ether, and dried over anhydrous sodium sulfate at reduced pressure to afford 0.397 g (66.2 % yield) of the hydroxo/ ethoxo compound.
Results and Discusson
The observation that platinum(IV) compounds might act as robust prodrugs for the delivery of effective platinum(II) chemotherapeutic agents has opened a wide range of possibilities for the formulation of antitumor drugs. In general, a platinum(II) compound is oxidized to a platinum(IV) species which may add ligands to generate compounds which display potential as antitumor agents or which provide reactive sites suitable for the attachement of a range of functionality [41,48]. In one approach, cis-dichloro(diammine) platinum(II) is oxidized with dinitrogen tetroxide in the presence of a suitable ligand (L) to produce a suitable platinum(IV) compound directly [48]. This is illustrated in Scheme 2.
This procedure seems to be unreliable and to lack generality for the convenient generation of useful platinum(IV) compounds. In a second, more useful, approach the platinum(II) compound is oxidized to generate the corresponding dihydroxy platinum(IV) compound. The hydroxyl groups are sufficiently nucleophilic to react with good electrophiles, most commonly succinic anhydride, to generate compounds with functionality that may be used for the attachment of a variety of groups [41].
The approach has been utilized for the attachment of both a carbon nanotube carrier and a folate targeting moiety [41]. All the compounds have been characterized using infrared spectroscopy and thermogravimetry. Infrared spectra are displayed in Figure 1. The depicted transformations are readily apparent from these spectra. Conversion of Cisplatin to the corresponding dihydroxo compound introduces a hydroxyl absorption at 3459 cm-1 into the spectrum. Transformation of the dihydroxo to the disuccinato compound generates a spectrum lacking the hydroxyl absorbtion but containing an ester carbonyl absorbtion.
Thermograms for the decomposition of these compounds are contained in Figure 2.
As can be seen all of the compounds undergo sequential ligand loss to afford a residue of platinum. For example, the dihydroxy intermediate displays an initial mass loss with an extrapolated onset temperature of 125°C corresponding to the loss of the hydroxyl ligands, followed by the loss of the ammonia ligands (233°C), and finally loss of the chloride ligands (321°C) to leave a residue of platinum. This is summarized in Table 1.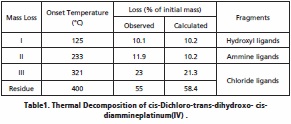
Conclusion
The thermal decomposition of several Pt(IV) precursors to antitumor drugs has been examined using thermogravimetry. In general, decomposition is well behaved and reflects sequential loss of ligands to generate a residue of platinum. Initial decomposition for cis-dichloro-trans-dihydroxy-cis-diamminoplatinum(IV) occurs at relatively low temperature (125oC) and reflects the loss of hydroxyl ligands. Conversion of the hydroxyl groups to succinate esters lead to a thermally more stable compound with initial decomposition corresponding to loss of the succinato ligands at 233oC.
References
1. Howell BA, Beholz LG, Sastry BBS. The thermal degradation characteristics of selected organoplatinum antitumor agents. J Therm Anal 1893;40:395-403.
2. Howell BA, Chhetri P, Dumitrascu A, Stanton KN. Thermal degradation of platinum(IV) precursors to antitumor drugs. J Therm Anal Cal 2009; submitted.
3. Rosenberg B, VanCamp L, Krigas T. Inhibition of cell division in Escherichia coli by electrolysis products from a platinum electrode. Nature 1965;205:698-6999.
4. Rosenberg B, VanCamp L, Grimley EB, Thomson AJ. The inhibition of growth or cell division in Escherichia coli by different ionic species of platinum(IV) complexes. J Biol Chem 1967;242: 1347-1352.
5. Rosenberg B, VanCamp L, Trosco JF, Mansour VH. Platinum compounds: a new class of potent antitumor agents. Nature 1969;222:385-386.
6. Rosenberg B. Platinum complexes for the treatment of cancer. In: Spiro TG, editor. Metal ions in biology, vol 1: Nuclei acid-metal ion interactions. New York: John Wiley and Sons; 1980. pp. 1-29.
7. Rosenberg B. Fundamental studies with cisplatin. Cancer 1985;55:2303-2314.
8. Jamieson ER, Lippard SJ. Structure, recognition, and processing of cisplatin-DNA adducts. Chem Rev 1999;99:2467-2498.
9. Wong E, Giandomenico CM. Current status of platinum-based antitumor drugs. Chem Rev 1999; 99:2451-2466.
10. Wang D, Lippard SJ. Cellular processing of platinum anticancer drugs. Nat Rev Drug Discovery 2005;4:307-320.
11. Dabrowiak JC, Bradner W. Platinum antitumor agents. Prog Med Chem 1987;24:129158.
12. Mistry P, Kelland LR, Loh SY, Abel G, Murrer BA, Harrap KR. Comparison of cellular accumulation and cytotoxicity of cisplatin with that of tetraplatin and amminedibutyratodichloro(cyclohexylamine) platinum(IV) (JM221) in human ovarian carcinoma cell lines. Cancer Res 1992;52(22):6188-93.
13. McKege M, Kelland L. New platinum drugs. In: Neidle S, Waring M, editors. Molecular aspects of drug-DNA interactions. New York: Macmillian; 1992. pp. 169-212.
14. Jones TW, Chopra S, Kaufman JS, Flamenbaum W, Trump BF. cis- Diammine-dichloroplatinum(II)-induced acute renal failure in the rat. Correlation of structural and functional alterations. Lab Invest 1985;52:363-374.
15. Rosenberg B. Biological effects of platinum compounds. New agents for the control of tumors. Platinum Metals Rev 1971;15(2):42-51.
16. Aggrawal SK, Menon GK. Ultrastructural localization of calcium(2+) and its possible role in the amelioration of kidney toxicity due to cisplatin. J Clin Hematol Oncol 1981;11:73-84.
17. Neuse E. Carrier-bound platinum and iron compounds with carcinostatic properties. Polym Adv Technol 1998; 9(10-11):786-793.
18. Lebwohl D, Canetta R. Clinical development of platinum complexes in cancer therapy: an historical perspective and an update. Eur J Cancer 1998;34:1522-1534.
19. Jagur-Grodzinski J. Polymers for targeted and/or sustained drug delivery. Polym Adv Technol 2009;20:595-606.
20. Hoste K, DeWinne K, Schacht E. Polymeric prodrugs. Int J Pharm 2004;277(1-2):119-131.
21. Khandare J, Minko T. Polymer-drug conjugates: Progress in polymeric prodrugs. Prog Polym Sci 2006;31(4):359-397.
22. Duncan R. Polymer conjugates as anticancer nanomedicines. Nat Rev Cancer 2006;6(9):688-701.
23. Duncan R. The dawning era of polymer therapeutics. Nat Rev Drug Discov 2003;2(5):347-360.
24. Batz H-G. Polymeric drugs. Adv Polym Sci 1977;23:25-53.
25. Langer R. Drug delivery and targeting. Nature 1998;392(6679, Suppl.):5-10.
26. Langer R. Drug delivery: Drugs on target. Sci 2001;293(5527):58-59.
27. Allen TM, Cullis PR. Drug delivery systems: Entering the mainstream. Sci 2004, 303(5665), 1818-1822.
28. Tomalia DA, Dewald JR, Hall M, Martin SJ, Smith PB. Preprint. 1st SPSJ International Polymer Conference, Kyoto, Japan 1984. p. 65.
29. Tomalia DA, Baker H, Dewald JR, Hall M, Kallos G, Martin S, Roeck J, Ryder J, Smith PB. A new class of polymers: starburst-dendritic macromolecules. Polym J 1985;17(1):117-132.
30. Tomalia DA. Starburst/cascade dendrimers: fundamental building blocks for a new nanoscopic chemistry set. Aldrichimica Acta 1993;26(4):91-101.
31. Tomalia DA. Frechet JMJ, editors. Dendrimers and Other Dendritic Polymers. New York: John Wiley and Sons Inc 2001.
32. Tomalia DA, Reyna LA. Dendrimers as multi-purpose nanodevices for oncology drug delivery and diagnostic imaging. Biochem Soc Trans 2007;35(1):61-67.
33. Tomalia DA. Birth of a new macromolecular architecture: Dendrimers as quantized building blocks for nanoscale synthetic organic chemistry. Aldrichimica Acta 2004;37(2):39-57.
34. Howell BA, Fan D, L Rakesh. Nanoscale dendrimer-platinum conjugates as multivalent antitumor drugs. In: Abd-El-Aziz AS, Carraher CE, Pittman CU, Zeldin M, editors. Inorganic and organometallic macromolecules: Design and applications. New York: Springer Sci; 2008, pp. 269-294.
35. Howell BA, Fan D. Poly(amidoamine) dendrimer-supported organoplatinum antitumor agents. Proc R Soc A 2009; in press.
36. Yang W, Pan C-Y. Synthesis and fluorescent properties of biodegradable hyperbranched poly(amido amine)s. Macromol Rapid Commun 2009;30:2096-2101.
37. Kim T-W, Chung PW, Slowing II, Tsunoda M, Yeung ES, Lin VSY. Structurally ordered mesoporous carbon nanoparticles as transmembrane delivery vehicle in human cancer cells. Nano Lett 2008;8(11):3724-3727.
38. Prato M, Kostarelos K, Bianco A. Functionalized carbon nanotubes in drug design and discovery. Acc Chem Res 2008;41(1):60-68.
39. Liu Z, Winters M, Holodniy M, Dai HJ. siRNA delivery into human T cells and primary cells with carbon-nanotube transporters. Angew Chem Int Ed 2007;46(12):2023-2027. 40. Liu Z, Cai W, He L, Nakayama N, Chen K, Sun X, Chen X, Dai H. In vivo biodistribution and highly efficient tumour targeting of carbon nanotubes in mice. Nature Nanotech 2007;2(1):47-52. 41. Dhar S, Liu Z, Thomale J, Dai H, Lippard SJ. Targeted single-wall carbon nanotube-mediated Pt(IV) prodrug delivery using folate as a homing device. J Am Chem Soc 2008;130(34):11467-11476.
42. Feazell RP, Nakayama-Ratchford N, Dai H, Lippard SJ. Soluble singlewalled carbon nanotubes as longboat delivery systems for platinum(IV) anticancer drug design. J Am Chem Soc 2007;129(27):8438-8439.
43. Dhar S, Weston DL, Giljohann DA, Mirkin CA, Lippard SJ. Polyvalent oligonucleotide gold nanoparticle conjugates as delivery vehicles for platinum(IV) warheads. J Am Chem Soc 2009;131:14652-14653.
44. Hall MD, Dillon CT, Zhang M, Beale P, Cai Z, Lai B, Stampfl APJ, Hambley TW. The cellular distribution and oxidation state of platinum(II) and platinum(IV) antitumour complexes in cancer cells. J Biol Inorg Chem 2003;8(7):726-732.
45. Ang WH, Pilet S, Scopelliti R, Buss F, Juilleavat-Jeannevet L, Dyson PJ. Synthesis and characterization of platinum(IV) anticancer drugs with functionalized aromatic carboxylate ligands: Influence of the ligands on drug efficacies and uptake. J Med Chem 2005;48(25):8060-8069.
46. Barnes KR, Kutikov A, Lippard SJ. Synthesis, characterization, and cytotoxicity of a series of estrogen-tethered platinum(IV) complexes. Chem Biol 2004;11(4):557-564.
47. Rieter WJ, Pott KM, Taylor KML, Lin W. Nanoscale coordination polymers for platinum-based anticancer drug delivery. J Am Chem Soc 2008;130(35):11584-11585.
48. Kay H, Palmer JW, Stanko JA. US Pat. 0080131 A1; 2005.
Bob A. Howell is currently Professor, Department of Chemistry, and Director, Center for Applications in Polymer Science at Central Michigan University. He has broad experience in the use of thermal methods of analysis, particularly as applied to polymer degradation and drug characterization. He is a past president of the North American Thermal Analysis Society and is currently a member of the Board of Directors. He is also a member of several prominent committees of the American Chemical Society and currently serves as Councilor from the Midland Section.
Adina Dumitrascu is a faculty research associate in the Department of Chemistry/ Center for Applications in Polymer Science at Central Michigan University. Her research interests include polymersupported organoplatinum antitumor agents, immobilized catalysts, nonhalogen environmentally-friendly flame retardants for polymeric materials, and stable membrane materials for proton exchange fuel cells.
Pratik Chhetri is an undergraduate at Central Michigan University completing B.S. thesis research in the area of platinum prodrugs and delivery systems.