Abstract
Development of a robust, reproducible biopharmaceutical manufacturing process is a critical path activity in the overall early development timeline. Platform processes have enabled companies to perform process development in shorter timeframes for molecules such as antibodies. However, technical challenges and regulatory requirements are more easily met by obtaining more process performance data during development and gaining a good understanding of critical process parameters. Today, multiple technologies and instruments are available to enable companies to develop early production processes on tight timelines while obtaining sufficient data to support process understanding. These high-throughput approaches to process development are described in this article.
Cell Culture Process Development and Optimization
Several instruments and technologies enable rapid development of a robust mammalian cell culture process prior to production of clinical material. Traditionally, cell culture development is initiated using small shake flasks. Once operating ranges are established, the process is transitioned to benchtop bioreactors for process confirmation. While shake flasks are important in cell culture development, they do not adequately simulate larger scale bioreactors regarding the monitoring and control of process parameters, including dissolved oxygen (DO) and pH. On the other hand, 1- to 5-L bioreactors do not enable high-throughput parameter screening, and they require significant resources to set up and monitor, although automated on-line systems such as the SegFlow®1 can facilitate monitoring. Therefore, it is often difficult or cost-prohibitive to run enough bioreactor experiments to define the critical process parameters fully and to develop a robust initial cell culture process.
Increasing pressure to reduce product development timelines, as well as expectations from the regulatory authorities for better process understanding, characterization, and validation,2 have resulted in the availability of more efficient process development tools. One approach is the use of high-throughput technologies that rely on either significantly scaled-down experimental methods or other miniaturized systems that can generate greater information about a unit operation in shorter periods of time while consuming less material.3-5 The successful application of high-throughput screening and mini-systems has been reported in many areas related to the development of biomanufacturing processes, such as clone screening and selection.6-8
Micro- and mini-bioreactors with working volumes of 0.7 mL to 500 mL that incorporate mixing, mass transfer, and control software comparable to a production bioreactor have been developed to allow cost- and time-efficient simulation of larger bioreactors. Today, many companies use miniaturized high-throughput cell culture development technologies to rapidly and efficiently define critical process parameters, followed by traditional laboratory-scale process development to confirm the details of individual unit operations. This approach can expand the total knowledge base on which a process is developed, thereby leading to a more robust and fully controllable manufacturing process.
One of the first high-throughput cell culture systems was the SimCell Micro Bioreactor,9 which used a mini-bioreactor card containing 6 individually controlled 700-μL chambers. Using robotics and associated automated controls, the SimCell could perform experiments using up to 1200 cell culture chambers in a single operation. However, the instrument was not widely adopted due to the high cost and the requirement to have specially trained operators to run the experiments. In addition, the throughput was considered almost too high to provide useful information for early development.
Pall Corporation offers the Micro-24 system that can run up to 24 simultaneous experiments with independent control and monitoring of each bioreactor’s gas supply, temperature, and pH. Each bioreactor has a working volume of up to 10 mL, providing a low-volume, high-throughput option for efficient cell culture development. The Micro-24 can be used for microbial fermentation or mammalian cell culture and is a cost-effective alternative to the SimCell product.
The Advanced Microscale Bioreactor (ambr™) from Sartorius Stedim Biotech is a newer micro-reactor product that has been widely implemented. The system offers parallel processing and evaluation in an automated workstation with 24 or 48 stirred-tank microbioreactors with working volumes of 10 mL to 15 mL. The bioreactors have individual monitoring and control of temperature, DO, and pH.10 Sartorius Stedim Biotech has also introduced the ambr250 system that provides for slightly larger working volumes of 100 mL to 250 mL.11
Intermediate scale systems such as the DASGIP Parallel Bioreactor System12 from Eppendorf contain multiple bioreactors in the range of 35 mL to 4 L that can be operated on a parallel basis with a single integrated controller. These larger bioreactors enable the assessment of gassing in the headspace, antifoam, and other parameters that are not accessible in the micro-reactor format. This system can integrate with analyzers such as cell counters and high-performance liquid chromatography, which is not available at the micro-reactor scale.
Another intermediate scale system called the Biopod is available from Fogale Nanotech.13 This system is capable of running 8 mini-bioreactors (with either 80 mL or 800 mL of working volume) at once. Additional systems for running multiple mini-bioreactors are also available from M2p-labs14 and other vendors. Some companies have also built their own high-throughput mini-bioreactor systems for process development.15 These systems are valuable for transitioning processes from the micro-reactor scale into a format that is fully transferable to production scale while still enabling some final evaluation and definition of critical process parameters.
Several publications highlight the usefulness and reliability of minibioreactor systems in cell culture development. In a study by Legmann, et al,16 data from 700-μL SimCell mini-bioreactors were compared to a 3-L bioreactor for the development and optimization of a cell culture process for an anti-interleukin-1 β monoclonal antibody (MAb). Excellent agreement was observed between the mini-bioreactor data and data from the conventional bioreactor. Regression analysis of the data showed that the correlation factors for each parameter measured were >0.84 and the product quality profiles were very similar.
Amanullah et al17 described the use of the SimCell for fed-batch cultivation of a glutamine synthetase (GS)-Chinese hamster ovary (CHO) cell expressing a model IgG4 MAb. Cell growth, process parameters, metabolic titer profiles, and protein titer profiles were compared to those from shake flask, benchtop, and pilot-scale bioreactor cultivations and found to be within +/-20% of the historical averages.
In another study, Hsu et al18 compared 4 recombinant CHO cell lines in a fed-batch process in the ambr™ system, shake flasks, and 2-L benchtop bioreactors. Cultures in ambr™ matched 2-L bioreactors in controlling the environment (temperature, DO, and pH) and in culture performance (growth, viability, glucose, lactate, Na(+), osmolality, titer, and product quality). Cultures in shake flasks did not show comparable performance to the ambr™ and 2-L bioreactors.
Rameez et al3 compared the ambr™ system to conventional bioreactor systems for their performance in MAb production in a CHO cell line. The ambr™ system was found to produce cell culture profiles that matched across scales of 3-L, 15-L, and 200-L stirred tank bioreactors. The processes used included complex feed formulations, perturbations, and strict process control within the design space, in-line with processes used for commercial-scale manufacturing of biopharmaceuticals. As Rameez et al3 noted, “Changes to important process parameters in ambr™ resulted in predictable cell growth, viability, and titer changes, which were in good agreement with data from the conventional larger scale bioreactors. Additionally, the miniature bioreactors were found to react well to perturbations in pH and DO through adjustments to the Proportional and Integral control loop.”
The data reported in these studies demonstrate the utility of high-throughput systems for cell culture development. They also demonstrate that conventional bioreactors can be adequately modeled using micro- or mini-bioreactors and that such systems allow for the investigation of culture conditions at greater statistical depth than can be performed in a conventional bioreactor. High-throughput cell culture technologies can be an effective tool for the development and optimization of cell culture processes as well as in troubleshooting cell culture problems, and their use will continue to increase.
Development and Optimization of Downstream Processes
High-throughput methods can be used to improve the speed and range of process development studies performed by studying a range of unit operations and process parameters with far less sample than traditional development normally requires. These high-throughput approaches rely on very small-scale chromatography columns or batch adsorption to evaluate chromatography resins, determine static and dynamic binding capacity, and evaluate a large variety of process parameters to aid in process optimization and the mapping of a purification design space.17
As shown in Table 1, many of the operating parameters of process scale columns can be investigated in parallel using either batch experiments in microtiter plates or micro-columns. For example, equilibrium constants and maximum (static) binding capacities of different chromatography media under different binding or elution conditions can be readily measured using microtiter plate-based highthroughput methods. For such batch adsorption studies, microtiter plates filled with chromatography media are used in conjunction with instrumentation to control the addition and removal of liquid from the wells. These plates can either be purchased already containing chromatography media from vendors such as GE Healthcare, Thermo Scientific, and Bio-Rad, but these suppliers only produce plates containing their chromatography media. As an alternative, empty microtiter plates can be filled in-house with any chromatography media but care must be taken and a reliable method must be used to ensure uniform distribution of the media and reproducibility.18 Microtiter plates are also available with ion exchange membranes fromPall Corporation and with ion exchange and hydrophobic membranes from Sartorius Stedim Biotech.
Table 1. Parameters to be Considered in Chromatography Step Development
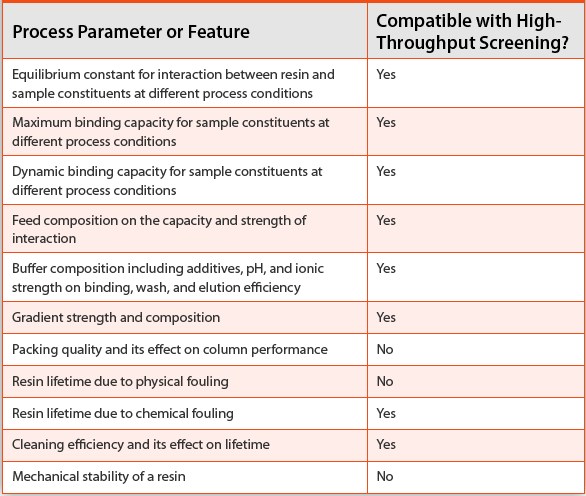
In general, information from batch adsorption studies is used to establish the media to be used for purification and the general binding and elution conditions for each chromatography. These broad design parameters can then be refined using micro-columns to fully define and characterize each unit operation. Once these have been established, the purification design and operating space can be verified using a qualified or validated manufacturing model. Atoll offers micro-columns packed with any commercially available chromatography media in sizes ranging from 50 μL to 200 μL packed with any resin. PhyNexus offers pipette tips in sizes of 10 μL to 500 μL pre-filled with a variety of chromatography media which can be used like micro-columns. Bio-Rad also offers 10-μL to 500-μL micro-columns. However, these columns are only available packed with Bio-Rad chromatography media. Any of these micro-columns can be connected to systems such as the Freedom EVO® system from Tecan19 or the PerkinElmer Janus® BioTX Pro, which allow several micro-columns, typically multiples of 8, to be run in parallel.
A qualitative comparison of the capabilities and attributes of different high-throughput formats is shown in Table 2.20 While microcolumns provide a more representative scale-down model for certain chromatography operations, microtiter plates have greater flexibility and lower cost for developing a chromatography unit operation.
Table 2. Comparison of High-Throughput Methods for the Development of Chromatographic Separations23
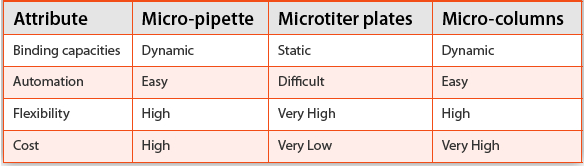
The Application of High-Throughput Technologies in Downstream Processing
Many examples of successful application of high-throughput techniques in downstream process development have been reported. These include investigations of a second step in the MAb purification process by cation exchange,21 weak partitioning,22 multimodal anion exchange,23 or hydrophobic interaction chromatography,24 screening of purification conditions,25 characterization of a multi-component adsorption system,26 estimation of dynamic binding capacities,27 and purification of virus -like particles.28
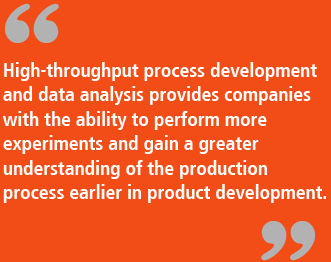
High-throughput methods have been used by a number of investigators for the development of chromatography steps for the MAb purification processes,29-35 as well as the development of non-chromatographic separations such as two-phase aqueous systems.36 For example, Lacki et al35 describe the use of microtiter plates to test different chromatography media and evaluate over 1000 different operating conditions in the development and optimization of an entire MAb purification process. This comprehensive study was completed in just 63 days by 1 scientist, demonstrating the efficiency of the highthroughput methodology.
For the initial capture step, Lacki et al35 studied the relationship between residence time and dynamic binding capacity to optimize the binding conditions for the antibody to MabSelect SuRe Protein A media, which was then verified in several small-scale column chromatography runs. In addition, the efficiency of different elution buffers was investigated using microtiter plates where it was found that the salt concentration of the elution buffer had no effect on either the overall step yield or monomer content of the recovered MAb. However, the overall yield and monomer content of the recovered MAb were affected by changes in the pH of the elution buffer. Decreases in pH increased the step yield up to 100% but also resulted in an increase in aggregate content.35
Following optimization of the Protein A capture step, aggregate removal by Capto Adhere media was evaluated using PreDictor plates from GE Healthcare. In an initial set of experiments, it was determined that both monomer and aggregates had bound to this media, making it impossible to obtain the desired yield and purity of the final product by running the column in a flow through mode. Therefore, the effect of salt type, salt concentration, and buffer pH on the efficiency of selective elution of monomer bound to the media was investigated, with conditions developed that allowed the recovery of monomeric antibody containing >1% aggregate. When these conditions were tested in a small-scale column, purified MAb containing >0.5% aggregate was recovered with a step yield of 87%.35
Summary
Recent technical advances have enabled a greater application of high-throughput methods to the traditionally labor-intensive activities involved in biopharmaceutical manufacturing process development. These include determining the optimal temperature, pH, and DO for cell culture processes or the best load and elute conditions for a chromatography column used to purify a biopharmaceutical. A variety of micro- and mini-reactor formats for cell culture and fermentation optimization, plate-based, and micro-column-based products for the development of purification processes and robotic liquid handling systems are available from multiple industry suppliers. Together with the ever-increasing computational power of today’s systems, these tools can result in the collection and analysis of very large data sets. High-throughput process development and data analysis provides companies with the ability to perform more experiments and gain a greater understanding of the production process earlier in product development. This emerging trend is aligned well with increasing regulatory expectations and with the principles of Quality by Design that are becoming more widely applied to biopharmaceutical development.
References
- Flownamics® Web site. Available at: http://www.flownamics.com/products_segflow.php. Accessed February 6, 2015.
- International Conference on Harmonisation of Technical Requirements for Registration of Pharmaceuticals for Human Use Expert Working Group (CH). Pharmaceutical Development Q8(R2) Step 4. Geneva: International Conference on Harmonisation of Technical Requirements for Registration of Pharmaceuticals for Human Use; 2009 Aug. 28 p. Available at: http://www.ich.org/fileadmin/Public_Web_Site/ICH_Products/Guidelines/ Quality/Q8_R1/Step4/Q8_R2_Guideline.pdf.
- Rameez S, Mostafa SS, Miller C, Shukla AA. High-throughput miniaturized bioreactors for cell culture process development: reproducibility, scalability, and control. Biotechnol Prog. 2014 May-Jun;30(3):718-727.
- Chhatre S, et al. A microscale approach for predicting the performance of chromatography columns used to recover therapeutic polyclonal antibodies. J Chomatogr A. 2009 Nov;1216:7806-7815.
- Long Q, et al. The development and application of high-throughput cultivation technology in bioprocess development. J Biotechnol. 2014 Dec;192 Pt B:323-338.
- Legmann R1, Benoit B, Fedechko RW, et al. A strategy for clone selection under different production conditions. Biotechnol Prog. 2011 May-Jun;27(3):757-765.
- Molecular Devices. Cell Screening and Bioproduction Web Site. Available at: http://www.moleculardevices.com/systems/cell-screening-and-bioproduction/clonepix-2-system. Accessed February 6, 2015.
- Sartorius Stedim Biotech. TAP Biosystems Key Applications Web Site. Available at: http://www.tapbiosystems.com/tap/applications/cell_line_selection.htm. Accessed February 6, 2015.
- iClick Media. Bioprocessors Web Site. Available at: http://www.iclickmedia.com/ bioprocessors/system.htm. Accessed February 6, 2015.
- Sartorius Stedim Biotech. Tap Biosystems Product Web Site. Available at: http://www.tapbiosystems.com/tap/cell_culture/ambr.htm. Accessed February 6, 2015.
- Sartorius Stedim Biotech. Tap Biosystems Product Web Site. Available at: http://www.tapbiosystems.com/tap/cell_culture/ambr_250.htm. Accessed February 6, 2015.
- Eppendorf. Benchtop Cell Culture Web Site. Available at: http://eshop.eppendorfna.com/ products/DASGIP_Parallel_Bioreactor_Cell_Culture_RD. Accessed February 6, 2015.
- Fogale Biotech Applications Web Site. Available at: http://www.fogalebiotech.com/PHP/applications-bioreactor.php. Accessed February 6, 2015.
- M2p-labs Micro Bioreactor Web Site. Available at: http://www.m2p-labs.com/products/ instruments. Accessed February 6, 2015.
- Cheung L, et al. Developing an automated high-throughput system for cell culture process development. Presented at International Business Communications Life Sciences’ BioProcess International Conference. 2008, Sep 22-26; Anaheim, CA.
- Legmann R, et al. A predictive high-throughput scale-down model of monoclonal antibody production in CHO cells. Biotechnol Bioeng. 2009;104(6):1107-20.
- Lacki KM, et al. High-throughput process development: determination of dynamic binding capacity using a microtiter plates filled with chromatography resins. Presented at the American Chemical Society’s 234th National Meeting. 2007 Aug 19-23; Boston, MA.
- Coffman JL, et al. High-throughput screening of chromatographic separations: I. Method development and column modeling. Biotechnol Bioeng. 2008 Jul 1;100(4):605-618.
- Tecan. Mannedorf (CH): Tecan Trading, AG; Freedom Evo® Overview; 2014. Available at: http://www.tecan.com/platform/apps/product/index.asp?MenuID=2694&ID=5270&Me nu=1&Item=21.1.8. Accessed February 6, 2015.
- Chhatre S, Titchener-Hooker NJ. Predicting process scale chromatographic performance using micro scale-down devices. Presented at University College of London’s Innovative Manufacturing Research Center, steering group meeting. 2008 Sep 17; London, United Kingdom.
- Kelley BD, et al. High-throughput screening of chromatographic separations: IV. Ion exchange. Biotechnol Bioeng. 2008;100(5):950-963.
- Kelley BD, et al. Weak partitioning chromatography for anion exchange purification of monoclonal antibodies. Biotechnol Bioeng. 2008;101(3):553-566.
- Lacki KM, et al. Accelerated development of a downstream purification process for production of monoclonal antibodies: a case study. Presented at the 13th Recovery of Biological Products Conference. 2008 Jun 22-27; Quebec City, Canada.
- Kramarczyk JF, et al. High-throughput screening of chromatographic separations: II. Hydrophobic interaction. Biotechnol Bioeng. 2008;100(4):707-720.
- Rege K, et al. High-throughput process development for recombinant protein purification. Biotechnol Bioeng. 2006;93(4):618-30.
- Linden T. Untersuchungen zum inneren transport bei der proteinadsorption an porose medien mittels konfokaler laser-rester-mikroskopie [Investigations of internal transport during protein adsorption on porous media using confocal laser scanning microscopy] [dissertation]. Dusseldorf (DE): Heinrich-Heine University Dusseldorf; 2001 Dec. Section 3.7.3, Einkomponentenadsorption monoklonaler antikorper oder BSA [Single component adsorption of monoclonal antibodies or BSA]; p. 87-88. German.
- Bergander T, et al. High-throughput process development: determination of dynamic binding capacity using microtiter filter plates filled with chromatography resin. Biotechnol Prog. 2008;24(3):632-639.
- Wenger MD, et al. An automated microscale chromatographic purification of virus-like particles as a strategy for process development. Biotechnol Appl Biochem. 2007;47(Pt 2):131-139.
- Bhambure R, et al. High-throughput process development for biopharmaceutical drug substances. Trends Biotechnol. 2011;29(3):127-135.
- Rodrigo G, Nilsson-Valimaa K. Developing a MAb aggregate removal step by highthroughput process development. BioPharm International. 2010;23(4):45-61.
- High-Throughput Process Development Conference. Extended reports from the second international conference on high-throughput process development; Avignon, France, 4-7 June 2012. Buckinghamshire (UK): GE Healthcare UK Ltd; 2012 Nov. 52 p. Available from: https:// www.gelifesciences.com/gehcls_images/GELS/Related%20Content/Files/1354036544405/ litdoc29034193_20140930090841.pdf.
- Chollangi S, et al. Accelerating purification process development of an early phase MAb with high-throughput automation. Part 1. Bioprocess Int. 2014;12(3): 48-52.
- Chollangi S, et al. Accelerating purification process development of an early phase MAb with high-throughput automation. Part 2. Bioprocess Int. 2014 Mar;12(4): 32-41.
- Wierling PS, et al. High-throughput screening of packed-bed chromatography coupled with SELDI-TOF MS analysis: monoclonal antibodies versus host cell protein. Biotechnol Bioeng. 2007;98(2):440-450.
- Lacki KM, et al. Development and scale-up of a downstream process for a monoclonal antibody: a case study. Presented at the Society for Biological Engineering’s Second International Conference on Accelerating Biopharmaceutical Development; 2009 Mar 9-12; Coronado, CA.
- Oelmeier SA, et al. Application of an aqueous two-phase systems high-throughput screening method to evaluate mAb HCP separation. Biotechnol Bioeng. 2011;108(1):69-81.
Author Biographies
Susan Dana Jones, PhD,Vice President and Principal Consultant with BioProcess Technology Consultants, is a seasoned biotechnology entrepreneur with experience in product development, outsourcing, and strategic planning. Dr. Jones is a subject matter expert in cell line development and characterization for biosimilar, new biopharmaceutical, and vaccine development programs. She has a broad knowledge of regulatory requirements for manufacturing products for human use and has prepared CMC sections of multiple regulatory submissions. She currently serves on the Board of Directors of Gene Solutions, the Scientific Advisory Board of Symphogen, and is a member of the Editorial Advisory Board of BioProcess International. She received her PhD in Genetics from the University of California, San Francisco.
Thomas C. Ransohoff, MS, Vice President and Principal Consultant at BioProcess Technology Consultants, is an expert in development and scale-up of biopharmaceutical processes, separations and purification technologies, cGMP manufacturing, and management of technologybased start-up businesses. He holds a BS in Chemical Engineering from MIT and an MS in Chemical Engineering from the University of California, Berkeley.
Frank Castillo, PhD, Senior Consultant with BioProcess Technology Consultants, has over 30 years of experience in the development and manufacturing of biopharmaceuticals with expertise in project management and process development for monoclonal antibodies, recombinant proteins, gene therapy vectors, and cell-based therapies. He holds a PhD in Microbiology from the College of Medicine and Dentistry of New Jersey.
Frank J. Riske, PhD, Senior Consultant at BioProcess Technology Consultants, has over 25 years of experience in the biopharmaceutical industry. Dr. Riske has extensive experience in the development of downstream processes for cytokines, proteins, and virus from plasma, E. coli, Pichia, and mammalian systems and in the development and manufacture of novel drug delivery systems. Dr. Riske received his BS in Biology from Fairfield University and a PhD in Biochemistry and Microbiology from Rutgers University, and completed a post-doctoral position at Hoffmann-LaRoche.
Howard L. Levine, PhD,President and Principal Consultant of BioProcess Technology Consultants and Managing Director of BPTC Europe, has over 30 years of experience in the development and commercialization of biopharmaceutical products. He has worked on products including vaccines, cell therapy, fusion proteins, antibodies, natural products, cytokines, and other recombinant proteins. Dr. Levine earned a PhD in Chemistry from the University of Chicago and completed a post-doctoral fellowship at Harvard University.