Introduction
In the last two years five novel fusion proteins were approved by the FDA, raising the total number of marketed therapeutic fusion proteins to thirteen. Several more are in late stage clinical trials and will probably reach the market soon. This underlines a success story that started in 1998 with the approval of Enbrel® the first fusion protein ever. Fusion proteins are generated by artificially joining two or more genes that originally code for separate proteins by genetic engineering. The result is a single polypeptide with functional properties of both parental proteins. These combinations of unrelated domains do not occur in nature and are solely based on intelligent human design.1 Often the components of fusion proteins are derived from different cell types or cellular locations that do not necessarily fit together in terms of physicochemical properties such as isoelectric point, charge densities and hydrophobicity which ultimately lead to manufacturing challenges. Here, the three areas, design, upstream and downstream processing, which contribute mostly both to the challenges and the respective solutions, are discussed (Figure 1).
Figure 1. Key factors for fusion protein manufacturing.Design
When designing fusion proteins the simple choice of the orientation of the individual modules can have a huge impact on functionality and production. This has been investigated in detail with some albumin or Fc-fusion proteins. In the case of Interferon the fusion partner albumin had to be placed at the C-terminus in order to avoid incomplete disulfide bridge formation and to increase stability.2 In contrast to most other Fc fusions, fibroblast growth factor 21 had to be connected to the C-terminus of the Fc domain to maintain its binding capability.3 Sometimes the reason for a certain preference is obvious, for instance when a free C- or N-terminus is required for activity. In other cases a certain orientation might surprisingly impact the expression level. This was observed for elastin like peptide (ELP) fusions, which were less active when the construct started with an ELP sequence at the amino terminus.4
Another important element is the linker joining both fragments. This stretch of amino acids can add functionalities, for instance a rigid linker could force a molecule into a desired spatial formation whereas a highly flexible linker might be required to enable a certain activity. Furthermore, the length of the peptide spacer is a variable of interest.5 Certain peptide sequences can be modified during the production process by glycosylation that protects from proteolysis. In the example of a glucagon-like peptide (GLP-1) antibody fusion, the clipping at the linker boundary by a host cell protease was observed which could be avoided if an O-glycosylation at a neighboring tyrosine shielded the protease sensitive site. This could be optimized by selecting a specific Chinese hamster ovary (CHO) cell line.6
However, a certain sensitivity to specific proteases could be desirable when fusion protein cleavage is required for activity. This was elegantly implemented in the design of a cleavable linker for the factor IX (FIX) albumin fusion protein. Here a sequence from one of the FIX sites was chosen that gets cleaved by activation factors of the coagulation cascade. Thus the half-life of the inactivated factor FIX is maintained while ensuring full activity and short half-life after cleavage without potential steric hindrance by albumin.7 In the design of fusion proteins at least one novel epitope is created at the conjunction between both fusion domains. Therefore it is necessary to assess the immunogenic properties of the whole fusion protein in order to avoid any unwanted immune response. Typical fusion protein design parameters are displayed in Figure 2.
Figure 2. Fusion protein design parameters.Upstream Processing
After defining the molecular design of the protein it needs to be expressed in production cell lines. The type of the heterologous fusion protein can have a strong influence on the selection of host organism. For instance, albumin fusions have been successfully expressed at very high product concentrations above 10 g/L in P. pastoris.8 Sometimes the co-expression of secretion-supporting co-factors can further improve the productivity.9 It is useful to evaluate different cell types because they might enable specific post-translational modifications or release different host cell proteins that could impact product quality and stability.6 One striking example is the reduction of disulfide bridges by components of the intracellular thioredoxin system liberated from lysed cells.10 In most cases Chinese hamster ovary (CHO) cells are the host of choice as significant knowledge exists about their physiology and in vitro cultivation which was acquired over long time.
As the typical fusion protein usually consists of only a single polypeptide chain, difficult titrations of gene constructs, as in the case of bispecific antibodies, can be avoided. More efforts are undertaken in upstream processing (USP) defining bioreactor and feed conditions such as galactose supplementation that affects sialylation which impacts plasma half life.11 In feeding studies of CHO cells expressing a cytokine trap fusion, galactose feeds seem to trigger high sialidase levels that negatively influence the degree of sialylation.12 When using hydrocortisone as additive, activating the glucocorticoid receptor (GR), both viability and sialylation are increased.13 Minimization of aggregation during cultivation could as well be achieved with hydrocortisone. Using dexamethasone (DEX) that also targets the GR induces the activity of glutathione reductase that is useful for disulfide bridge containing fusion proteins.14 Additionally DEX can also enhance the sialylation through induction of sialyltransferases.15 Another option to improve the sialic acid content is the supplementation with LiCl which also increases specific productivity of CHO cells.16 Further effects on the sialylation were demonstrated when replacing glutamine by α-ketoglutarate in an effort to reduce the deleterious accumulation of ammonia in the culture medium.17 Glutamine-free cultivation can also be the method of choice to drive growth, productivity and glycosylation in the case of an Fc-erythropoietin fusion protein.18 The various approaches to influence sialylation are summarized in Table 1. Productivity cannot only be influenced by additives but also through adaptations in the cultivation process. For instance, by switching in a biphasic process from conditions initially supporting rapid growth to generate a high cell mass to conditions allowing maximum specific protein concentration by reducing the cultivation temperature, higher productivity and less aggregation for a Fc fusion protein was acheived.19 The positive effects of lower temperature (prolonged viability, reduced metabolism, increased specific productivity) can be combined with the impact of lower pH (prolonged viability, reduced metabolism, unaffected sialylation) in a synergistic way to maximize the protein yield.20 When scaling up cultivation, technical parameters such as dissolved oxygen must be taken into account, otherwise oxidative stress might lead to altered glycosylation profiles.21
Table 1. Approaches to influence sialysation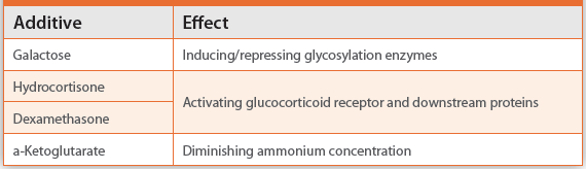
Downstream Processing
On the first glance Fc-fusion proteins should be at least amenable to the arsenal of platform downstream processes developed for antibodies. Interestingly, it was found out that dynamic binding capacities of protein A resins did not correlate to the molecular size of fusion proteins. One reason is the lack of variable domains in Fc-fusions that usually would contribute to protein A binding of antibodies. The second reason for lower capacity is the steric hindrance of Fc-fusions due to their larger apparent size.22 Albumin fusions are the other class of molecules for which affinity-based resins for capture are available. Initially, blue dye ligands were used, but currently either a synthetic ligand based on a triazine scaffold is available,23 or alternatively capture through Camelid-derived single domain antibody fragments can be done.
During downstream processing (DSP) of fusion proteins aggregation is a frequently occurring complication. Aggregates in the drug product can elicit unwanted immune responses or interfere with assays resulting in wrong potencies.24 Sometimes Fc-fusion proteins aggregate during low pH elution from a protein A column. If the structure of the fusion protein is destabilized during the binding to the resin, the formation of aggregates can be suppressed by the addition of low urea concentrations.25 If an acidic pH induces aggregate formation, an alkaline pH could be used instead to promote elution from a protein A column.26 Sensitivity to acidic pH excludes the use of low pH for virus inactivation. Therefore alternative methods such as virus inactivation by detergents or organic solvents must be employed. Aggregates of structurally instable fusion proteins can be induced during elution from ion exchangers at low pH and high salt conditions as well. This could be diminished by lowering load concentration and switching to a less hydrophobic resin.27 With respect to pH-dependent conformational stabilities, actions should be taken to support the least stable domain as shown for abatacept.28 Although in most cases excipients like sucrose stabilize protein solutions, it cannot be ruled out that under mechanical stress they might promote aggregate formation by not maintaining colloidal stability as for an interferon albumin fusion protein that additionally loses its conformational stability at lower pH.29
One cause for product-related impurities can be disulfide scrambling, particularly if both fusion partners contain cysteines as described for instance for an Fc fusion protein. Interestingly, the disulfide scrambling did not affect the Fc-part but was dependent on the glycosylation pattern.30 Sometimes even the mutagenesis and rearrangement of cysteine residues must be applied to reduce aggregate formation and to stabilize the functional conformation.31 This is even more important if disulfide mispairing is induced by a free cysteine whose elimination solves the issue.32 Free thiol groups can also contribute to aggregate formation during freezing. Again, the Fc-domain was unaffected, as mispairing was only observed in the fusion partner domain. Freezing increases the local concentration of protein, which might further increase due to salting out effects when too much NaCl is present. The situation gets more complicated if a temperature-sensitive buffer substance such as MOPS is used that increases pH at lower temperatures.33 The different causes for aggregation are summarized in Table 2.
Table 2. Causes for aggregation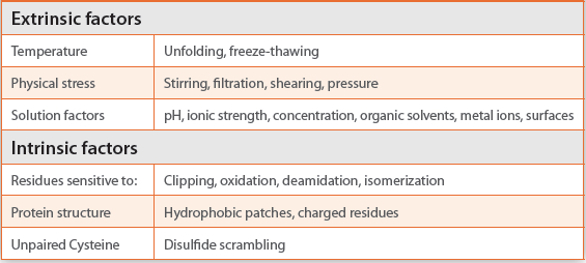
Conclusion
Fusion proteins are a highly interesting class of therapeutic molecules, as they can be designed to fulfil new modes of action, can be produced as a single molecule, allow equal biodistribution of two functionalities and are often used in the context of biobetters with improved pharmacokinetic and pharmacodynamic values. When evaluating manufacturing issues it becomes apparent, that they are often related to flaws in the design and require more process development efforts to optimize quality attributes such as glycosylation. Frequently they do not express as well as some of their individual modules they are derived from. In DSP a key challenge is the suppression or removal of aggregate species that could trigger unwanted effects in patients. But in the last two decades much progress has been achieved in understanding the underlying reasons and finding solutions to solve these challenges.
References
- Schmidt, S. R. in Fusion Protein Technologies for Biopharmaceuticals: Applications and Challenges (ed. Schmidt, S. R.) 3-24 (Wiley, 2013).
- Zhao, H. L. et al. J. Biotechnol. 131, 245–52 (2007).
- Hecht, R. et al. PLoS One 7, e49345 (2012).
- Christensen, T. et al. Protein Sci. 18, 1377–87 (2009).
- Chen, X., et al. Adv. Drug Deliv. Rev. 65, 1357–69 (2013).
- Dorai, H. et al. Biotechnol. Bioeng. 103, 162–76 (2009).
- Metzner, H. J. et al. Thromb. Haemost. 102, 634–644 (2009).
- http://www.vtu-technology.com/Albumins-&-albumin-fusion-proteins/en/2031/l/516
- Guan, B. et al. Yeast 33, 587–600 (2016).
- Hutterer, K. M. et al. MAbs 5, 608–613 (2013).
- Liu, J. et al. World J Microbiol Biotechnol 31, 1147–56 (2015).
- Clark, K. J. R. et al. Biotechnol. Bioeng. 90, 568–577 (2005).
- Rouiller, Y. et al. Biotechnol. Prog. 28, 803–813 (2012).
- Qian, Y. et al. Biotechnol. Prog. 26, 1417–23 (2010).
- Jing, Y. et al. Biotechnol. Bioeng. 107, 488–96 (2010).
- Ha, T. K. et al. Appl. Microbiol. Biotechnol. 98, 9239–48 (2014).
- Ha, T. K. et al. J. Biotechnol. 180, 23–29 (2014).
- Taschwer, M. et al. J. Biotechnol. 157, 295–303 (2012).
- Kaisermayer, C. et al. J. Biotechnol. 227, 3–9 (2016).
- Trummer, E. et al. Biotechnol. Bioeng. 94, 1033–1044 (2006).
- Lewis, A. M. et al. PLoS One 11, 1–24 (2016).
- Ghose, S. et al. Biotechnol. Bioeng. 96, 768–779 (2007).
- Williams, S. et al. Methods Mol. Biol. 1129, 181–95 (2014).
- Bajardi-Taccioli, A. et al. Mol. Immunol. 67, 616–624 (2015).
- Shukla, A. et al. J. Chromatogr. A 1171, 22–8 (2007).
- Rea, D. W. et al. Biopharm Int. 20-25 (2008).
- Chen, Z. et al. J. Chromatogr. A 1460, 110–122 (2016).
- Fast, J. L. et al. Biochemistry 48, 11724–36 (2009).
- Chou, D. K. et al. J. Pharm. Sci. 101, 2702–2719 (2012).
- Strand, J. et al. J. Pharm. Sci. 102, 441–453 (2013).
- Way, J. C. et al. Protein Eng. Des. Sel. 18, 111–118 (2005).
- Zhao, H. L. et al. Eur. J. Pharm. Biopharm. 72, 405–411 (2009).
- Wang, T. et al. J. Pharm. Biomed. Anal. 72, 59–64 (2013).
Author Biography
Dr. Stefan R. Schmidt currently serves as Senior Vice President for Process Science and Production at Rentschler Biotechnology. Previously he held various senior management positions in international Biotech and Pharma Corporations. In the last 10 years he worked intensively with fusion proteins, just recently editing and authoring the first comprehensive book on that topic.