Introduction
Reliable, consistent, predictable and cost-effective protein purification platforms, with the smallest possible number of steps, are highly desirable for protein research and manufacturing. This is one of the reasons why intervening protein (intein)-based protein purifications have become a focal point among other intein applications. Knowledge of inteins has expanded exponentially over the last three decades, resulting in many new patents and publications, as well as the identification of inteins in archaea (47%), bacteria (25%), hages, viruses, and even single and multi-celled eukaryotes.1 Additionally, inteins have now been artificially modified and fused to different functional protein domains to improve their utility in protein purification systems. Existing intein-based purification systems currently use three distinct types of inteins: full-length inteins that contain a splicing domain and an endonuclease domain, mini-inteins that contain a splicing domain but lack an endonuclease domain, and split-inteins that consist of two short intein segments (the N-terminal intein, IN, and C-terminal intein, IC), which must reassemble in order to splice. To develop these systems, inteins have been engineered with increasing functionality over the last several decades, leading to increasingly useful purification platforms. Early inteins possessed simple mutations to interrupt the native splicing reaction, which led to isolated cleaving activities, but they were slow and unreliable, and required problematic reagents. Later designs increased the cleavage rates and control, but were then plagued with premature cleaving and a persistent lack of reliability. A most recent design seems to have solved these problems; providing highly effective control and reliability, along with simple and inexpensive reagents. The major steps in developing these systems, along with some of the early efforts are herein described.
Importance of Intein/Extein Junction Mutations for Generating Isolated Cleaving Activities
Early work established that intein-mediated protein splicing is a spontaneous process, where the intein excises itself from between two flanking extein segments in a self-catalyzed splicing reaction. Key to the reaction are a highly conserved N-terminal Cysteine, Serine or Threonine, along with a C-terminal Asparagine or Glutamine on the intein sequence, which are directly involved in the bond rearrangements of the canonical splicing reaction.2-5 The only required residue external to the intein is a conserved Serine or Cysteine at the N-terminus of the C-extein (although this residue is not required for cleaving). During the splicing process, the intein/extein peptide bonds flanking the intein are cleaved as the N- and C-exteins are ligated, resulting in ejection of intein and formation of the mature host protein consisting of the ligated exteins.
Over time it was discovered that the intein/extein junction residues could be modified by a single Cysteine to Alanine or Asparagine to Alanine mutation, which would suppress the intein splicing activity while allowing residual C- or N- terminal self-cleavage side reactions, respectively. At the same time, research showed that the resulting cleavage rates could be controlled simply by changing the pH and/or temperature, or by adding of thiol-containing compounds.6-9
Thiol and Disulfide (S-S) Bond Breakage
The first commercially available intein-based protein purification systems were known as the IMPACTTM and IMPACT-CNTM systems (Intein Mediated Purification with an Affinity Chitin-binding Tag). These systems relied on a modified full-length intein (454 amino acid residues) derived from the Saccharomyces cerevisiae VMA1 gene. This system is still in use, although the cleavage reaction requires a high concentration of thiol (e.g. 1, 4-dithiothreitol, β-mercaptoethanol or cysteine), which limits the scalability of the process. Perhaps most importantly, the required thiol (or other reducing agent) affects disulfide bonds in the target proteins, which often results in unwanted target degradation and yield loss. Additionally, this bulky intein was often larger than the target proteins, and exhibited variable cleavage rates in the laboratory.
A pH Sensitive Intein Development as a Thiol Replacement
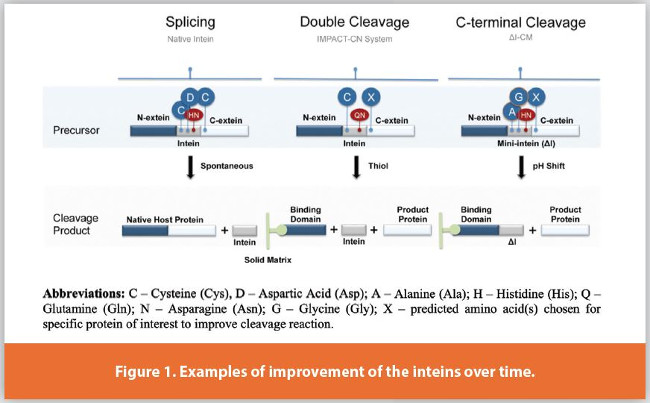
For broader industrial and laboratory applications, additional inteins were developed where the cleavage reaction was controlled with a small pH shift. These inteins were specifically designed to be benign to proteins with disulfide bonds, and be cost-effective through the use of simple buffers. An early example was the ΔI-CM mini-intein (referring to a Cleavage Mutant minimal intein), which was developed from the Mycobacterium tuberculosis RecA intein. To develop this intein, the native endonuclease domain was first deleted from the full-length parent intein, then the initial intein Cysteine was mutated to Alanine to suppress splicing, and finally an internal Aspartic Acid to Glycine mutation was identified to accelerate cleaving.8 These mutations provided accelerated C-terminal cleavage at pH 6.2, but suppressed cleavage at pH 8.5, when compared to both the initially designed mini-intein and full-length parent (see Figure 1). This intein is highly effective for most simple proteins expressed in E. coli, but often exhibits premature cleaving of targets during expression. Critically, premature cleaving by this intein has effectively limited its used to proteins expressed in the E. coli cytoplasm, thus necessitating a new intein for mammalian and other secreted protein systems.
Subscribe to our e-Newsletters
Stay up to date with the latest news, articles, and events. Plus, get special offers
from American Pharmaceutical Review – all delivered right to your inbox! Sign up now!
Development of a Split-Intein Protein Purification Platform
A Split Intein as a Possible Solution to Premature Cleavage Problems
During early mechanistic studies, contiguous inteins were sometimes artificially split into two segments to control splicing and cleaving reactions. Although this potentially provided a new means for suppressing premature cleaving, the split intein segments were often difficult to express and reassemble without a refolding step. Soon thereafter were found examples of naturally split inteins, where two intein segments fused to their respective exteins are expressed, assemble and splice inside their host cell in a process referred to as trans-splicing. The naturally occurring split inteins expressed and folded well, and were quickly adopted for protein purification methods. Although no premature cleaving was observed, control of the cleaving reaction in the assembled intein was still lacking. In one early system, thiol was shown to induce rapid cleavage, but reliability and the effects on disulfide bonds was still a concern.10 Further, this and other inteins often required three amino acids to be added to the terminus of the desired target protein in order to guarantee reliable cleaving, which was unacceptable in cases where a truly native target was required.
A Breakthrough in Split Intein Development
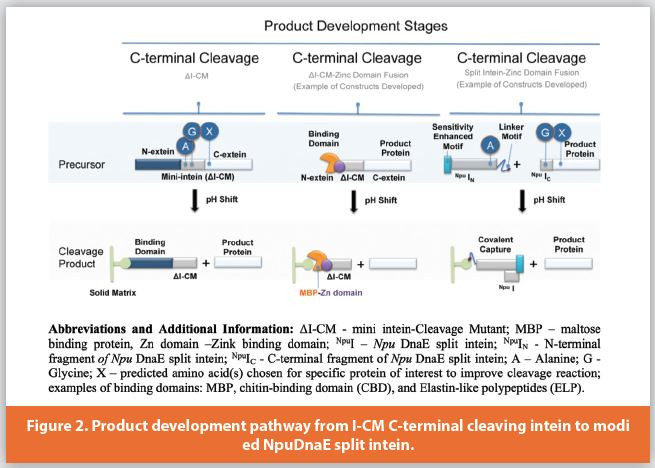
Led by previous work in developing the contiguous ΔI-CM intein (US Patent #6,933,362), highly specific mutations were rationally introduced into the naturally split Npu DnaE intein. Although the resulting intein resolves premature cleavage issues and provides somewhat accelerated cleaving, the ability to control cleaving via pH required additional development. This advance was provided through a rationally designed sensitivity-enhancing domain, which was originally intended to increase sensitivity to zinc, but had the additional effect of increasing pH sensitivity in the absence of zinc (US Patent #9,796,967).11,12 The resulting intein exhibits highly pH sensitive cleaving, while resolving premature cleaving and providing rapid cleaving after purification. Importantly, additional research on this intein has provided guidance on designing reliable or at least predictable performance of the cleaving reaction with various target proteins, effectively eliminating the need for native extein residues to be added to the target protein. These advances ultimately led to the development of a new purification platform, consisting of a capture resin with an immobilized N-terminal segment of a modified Npu DnaE split intein, which captures the small C-terminal intein segment appended to an expressed target protein (US Patent #10,066,027, issued in September, 2018).13 Once commercialized, the user would simply tag their target protein with the 36 amino acid C-terminal intein segment, express the resulting fusion in the host of their choice, bind the tagged target to the capture resin, wash away impurities with a pH 8.5 buffer, and then cleave the target from the immobilized tag with a buffer shift to pH 6.2. Importantly, stripping the cleaved tag in a subsequent process can then be done to regenerate the resin. A simplified illustration of this protein purification method is described in Figures 2 and 3.
On a side note, it is interesting that even though the pH-sensitive inteins described here are derived from different species (the ΔI-CM mini-intein was originally derived from the Mycobacterium tuberculosis RecA intein, while the split intein was derived from the Nostoc punctiforme DnaE intein), the gene mutations were “transferable” in terms of their functional impact on behavior of the intein.10 This newly patented split Npu DnaE intein performs similarly to the earlier ΔI-CM intein in terms of pH sensitivity and general cleaving rate, where both purifications take place at pH 8.5 followed by a pH 6.2 cleaving reaction. The key difference is the suppression of premature cleaving by splitting the intein during expression, and the now predictable performance of the cleaving reaction with different target proteins. In practice, the system is comparable to the classic FLAG Tag affinity method in terms of achieving a high-purity target protein, but with the added advantages of a tagless target and more affordable purchase and operating costs.
Conclusions
Over the last 15 years, intein purification systems have been tested with proteins expressed in a wide variety of host cells, including bacteria, yeast, plants and mammalian cells, as well as with different conventional and non-chromatographic purification tags (e.g., Elastin-Like Peptide, olyhydroxybutyrate-binding phasins and the self-aggregating peptide fusion tags 18A and ELK16).8,14-22 The advantage of these methods is that the target protein can be released from effectively any purification tag without the additional step of proteolytic tag removal. In the split intein system, the resin effectively becomes part of the intein cleaving process, providing a strong and selective purification with tag removal functionality in a single operation. This unique technology potentially provides a completely new and disruptive method for producing therapeutic proteins at research and manufacturing scale, and it is projected that it will ultimately constitute a core approach for industry.
It is predicted that the introduction of this technology to the market will have a great impact on how biopharmaceuticals are discovered, developed and manufactured, and will introduce new flexibility and simplicity compared to the existing complex, conventional methods. This technology also brings a truly groundbreaking opportunity to simplify and accelerate biopharmaceutical research, which equates to more rapid development of therapeutics that can save and prolong patient lives.
Author Biographies
Izabela Gierach, PhD, MBA, MS is a co-founder, CEO of Protein Capture Science, with over 17 years of professional experience in scientific research (including research on intein systems, non-clinical and clinical research), business project management, and products development.
David Wood, PhD is a Professor of Chemical and Biomolecular Engineering at The Ohio State University and co-founder, CSO of Protein Capture Science, with biopharmaceutical experience at Amgen. He has developed the intein technology described herein.
References
- Lennon CW, Belfort M. Inteins. Curr Biol. 2017;27(6):R204-R206.
- Li Y. Split-inteins and their bioapplications. Biotechnol Lett. 2015;37(11):2121-2137.
- Cheriyan M, Perler FB. Protein splicing: A versatile tool for drug discovery. Adv Drug Deliv Rev. 2009;61(11):899-907.
- Cooper AA, Stevens TH. Protein splicing: self-splicing of genetically mobile elements at the protein level. Trends Biochem Sci. 1995;20(9):351-356.
- Hirata R, Ohsumk Y, Nakano A, Kawasaki H, Suzuki K, Anraku Y. Molecular structure of a gene, VMA1, encoding the catalytic subunit of H(+)-translocating adenosine triphosphatase from vacuolar membranes of Saccharomyces cerevisiae. J Biol Chem. 1990;265(12):6726-6733.
- Shah NH, Muir TW. Inteins: Nature’s Gift to Protein Chemists. Chem Sci. 2014;5(1):446-461.
- Southworth MW, Amaya K, Evans TC, Xu MQ, Perler FB. Purification of proteins fused to either the amino or carboxy terminus of the Mycobacterium xenopi gyrase A intein. Biotechniques. 1999;27(1):110-114, 116, 118-120.
- Wood DW, Wu W, Belfort G, Derbyshire V, Belfort M. A genetic system yields self-cleaving inteins for bioseparations. Nature biotechnology. 1999;17(9):889-892.
- Lahiry A, Fan Y, Stimple SD, Raith M, Wood DW. Inteins as tools for tagless and traceless protein purification. Journal of Chemical Technology and Biotechnology. 2017;93(7):1827-1835.
- Guan D, Ramirez M, Chen Z. Split intein mediated ultra-rapid purification of tagless protein (SIRP). Biotechnology and bioengineering. 2013;110(9):2471-2481.
- Belfort M, Belfort G, Derbyshire V, Wood DW, Wu W, Inventors; Rensselaer Polytechnic Institute, assignee. Genetic system and self-cleaving inteins derived therefrom, bioseparations and protein purification employing same, and methods for determining critical, generalizable amino acid residues for varying intein activity. US patent 6,933,362. August 24, 2005.
- Ma B, Nellis DF, Zhu JS, Wood DW, Inventors. Compositions related to controllable intervening protein sequences (CIPS) comprising reversible zinc-binding motifs and inteins. US patent 9,796,967. October 24, 2017.
- Wood DW, Shi C, Inventors; Ohio State Innovation Foundation, assignee. Protein Production Systems and Methods Thereof. US patent 10,066,027. September 4, 2018.
- Fong BA, Gillies AR, Ghazi I, et al. Purification of Escherichia coli RNA polymerase using a self-cleaving elastin-like polypeptide tag. Protein science: a publication of the Protein Society. 2010;19(6):1243-1252.
- Fong BA, Wood DW. Expression and purification of ELP-intein-tagged target proteins in high cell density E. coli fermentation. Microbial cell factories. 2010; 9:77.
- Gillies AR, Hsii JF, Oak S, Wood DW. Rapid cloning and purification of proteins: gateway vectors for protein purification by self-cleaving tags. Biotechnology and bioengineering. 2008;101(2):229-240.
- Wu WY, Gillies AR, Hsii JF, et al. Self-cleaving purification tags re-engineered for rapid Topo(R) cloning. Biotechnology progress. 2010;26(5):1205-1212.
- Wu WY, Miller KD, Coolbaugh M, Wood DW. Intein-mediated one-step purification of Escherichia coli secreted human antibody fragments. Protein expression and purification. 2011;76(2):221-228.
- Shi C, Meng Q, Wood DW. A dual ELP-tagged split intein system for non-chromatographic recombinant protein purification. Applied microbiology and biotechnology. 2013;97(2):829-835.
- Warren TD, Coolbaugh MJ, Wood DW. Ligation-independent cloning and self-cleaving intein as a tool for high-throughput protein purification. Protein expression and purification. 2013;91(2):169-174.
- Fan Y, Miozzi JM, Stimple SD, Han T-C, Wood DW. Column-Free Purification Methods for Recombinant Proteins Using Self-Cleaving Aggregating Tags. Polymers. 2018;10(5):468/461-468/412.
- Shi C, Han TC, Wood DW. Purification of Microbially Expressed Recombinant Proteins via a Dual ELP Split Intein System. Methods Mol Biol. 2017;1495:13-25.