Abstract
Freeze-drying is a popular method of prolonging the shelf life of protein drug products, but lyophilization cycles can be long and expensive. Normally amorphous and partially crystalline protein formulations are kept below their collapse temperature during primary drying, but there has been recent interest in maintaining product temperature well above the collapse temperature even though the cake is transiently subject to viscous flow. This elevation of the product temperature can reduce the cycle time by one half or more but the impact of this action on the quality of the resulting drug product is an open question The basic phenomenon of collapse, both micro and macro, is described and several issues surrounding collapse are identified and discussed. The majority of the investigations have found little or no decrease in product quality but a few studies have found some decrease in long-term stability.
Background
Freezing the typical protein formulation results in two phases: about 80% of the frozen solution consists of crystals of pure ice and the remaining 20% is an amorphous or partially crystalline phase that contains all the excipients and protein (concentrated about 5X) along with some amorphous water left behind after the ice crystals have formed It is this amorphous or partially crystalline phase that ends up as a honeycombed cake at the end of the freeze-drying cycle with pores where the crystals of pure ice had been. The traditional approach to the design of a lyo cycle is to maintain this completely or partially amorphous phase in a glassy state during primary drying which involves keeping the temperature of the newly freeze-dried material below Tg’, the glass-rubber transition temperature of the amorphous phase which still contains about 20% water. For sucrose-based formulations Tg’ is -34°C to -25°C depending on the protein content Above this temperature the material becomes rubbery and is temporarily subject to viscous flow until the water content drops to a lower level. If there is too much viscous flow during primary and secondary drying, the pores could collapse and greatly reduce the flow of water vapor through the dry layer as well as result in an unacceptable product [3].
However, it has been demonstrated that there can be a situation, referred to as microcollapse, in which the basic pore structure of the dry layer remains intact even though the product temperature is well above Tg’ during primary drying and the amorphous phase is subject to some viscous flow. If there is loss of the pore structure, that would then be referred to as “macrocollapse” [4]. There are two basic ways of controlling the degree of collapse:
- In partially crystalline formulations, crystals of mannitol or other excipient are formed which provide the basic structural framework for the cake which is maintained even if the amorphous portion is above its Tg’ and is subject to viscous flow. Generally a ratio of 4 parts crystalline component (often mannitol or glycine) to 1 part amorphous component (often sucrose or trehalose) will result in a “microcollapse” situation [5]. As this ratio is reduced the degree of visual collapse increases until at a ratio of 1 to 1 or less the mannitol or glycine will fail to crystallize [6-7].
- Completely amorphous formulations containing 20 mg/ mL protein or more are not prone to “macrocollapse” [2,8-9]. A 50 mg/mL protein formulation becomes 250 mg/mL when concentrated by a factor of five and reaches a point where the viscosity increases dramatically and is about 100 times more viscous than a sugar solution of the same wt. % concentration. Apparently, viscous flow is reduced dramatically in these formulations. Placebos and low protein formulations can also exhibit “microcollapse” as long as the product temperature is kept only a degree or two above Tg’ by choosing an appropriate shelf temperature [10-12].
Table 1- Some Issues and Concerns about the Collapsed State
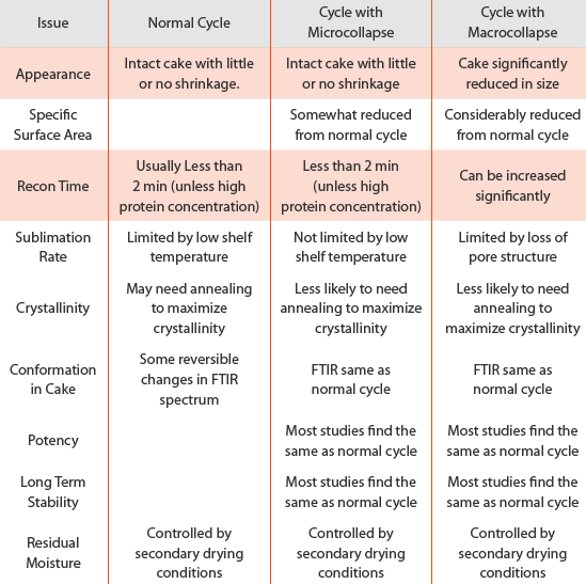
Table 2-Some Recent Studies that Examine Effects of Micro and/or Macrocollapse
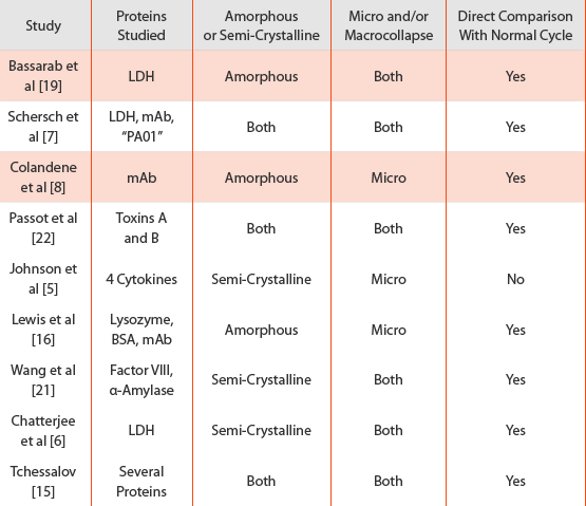
Product Quality Concerns and Issues: Current Status
The potential advantage of freeze-drying above the collapse temperature during primary drying have long been appreciated: the higher shelf temperatures and sublimation rates can reduce the cycle time by half or more. Until recently, however, the collapsed state has been avoided because of fears that the product quality could be adversely affected. Some of the issues that have been raised (Table 1) include appearance, specific surface area, sublimation rate, residual moisture, reconstitution time, physical state, and stability over time. Some pertinent data from the literature are listed in Table 2.
Appearance
The most obvious issue is the visual “collapse” of the cake which in this review is referred to as “macrocollapse”. Beyond any damage to the API itself, the shrunken appearance of a collapsed cake leads to a loss of pharmaceutical “elegance” and may put it at a commercial disadvantage to products with cakes that do not shrink. Recent studies, however, have demonstrated that, in most cases, the cake can be maintained in a “microcollapsed” state where visual appearance is not an issue. This is especially true for protein concentrations above 10 or 20 mg/mL. The increasing popularity of spray drying and foam drying may reduce the importance of cake appearance [13].
Specific Surface Area
The degree of collapse can be most accurately established by the measurement of the specific surface area of the lyophilized material [7,13]. As noted above, changing the ratio of crystalline versus non-crystalline excipient can generate cakes with varying degrees of collapse when dried above Tg’ and has been used as a tool to investigate the effect of cake collapse on product quality [6].
Sublimation Rate
A related issue is the sublimation rate during primary drying. The efficient removal of water vapor from the sublimation front requires that the pore structure of the dry layer remain intact. Once again, it has been shown that the apparent product resistance of the dry layer is maintained or even reduced if the newly freeze-dried material is maintained in the microcollapsed state. In fact, the path taken by water molecules during primary drying in the microcollapsed state appears to be less tortuous and does not present a significant barrier to the transit of water molecules across the dry layer [2,14].
Residual Moisture and Recon Time
A collapsed cake with little or no pore structure could take an excessive time to reconstitute and/or be resistant to drying during secondary drying. Cakes that have been kept in the microcollapsed state, however, have been shown to have the same reconstitution time and residual moisture as cakes that have been kept below Tg’ during primary drying [15-16]. Even cakes that exhibit some degree of “macrocollapse” have been shown to have the same moisture levels, recon times and Tg (the glass rubber transition temperature of the lyophilized cake) as “normal” cakes [7,15] despite concerns that acceptable levels of moisture may not be achievable [4].
Physical State of Protein and Excipients in the Cake
There have been at least two areas of concern about the physical state of cakes that have spent some time in the rubbery state during the lyo cycle
Crystallinity
One issue is the degree of crystallinity of semi-crystalline formulations. It is possible that, much like an annealing step, the degree of crystallinity of an excipient such as mannitol or glycine could increase while it is in the rubbery state [7]. Normally it is desirable to maximize the crystallinity so that it will not change on long term storage so this may not be an area of major concern.
Protein Aggregation, Changes in Conformation and Bioactivity
Another issue that has been mentioned is the possibility of the partial unfolding of protein molecules either due to the basic cold denaturation of proteins or to the transient exposure to an ice or mannitol crystal surface. There is considerable evidence, primarily from FTIR [17], that proteins in lyophilized cakes have a somewhat modified secondary structure, though this modification usually disappears upon reconstitution. If the molecules spend an extended period of time in a partially unfolded state while in the rubbery state during primary and secondary drying, it could lead to an increase in high molecular weight species or particles. This possibility was recently investigated for both a mAb and a non-mAb protein and no significant differences in high molecular weight species were found between conventionally and aggressively freeze-dried proteins [7,16]. Other studies [8,14,16] have found that mAb’s and non-mAb’s that had been freeze-dried while in the microcollapse state exhibited no detectable changes in IR spectrum. Chatterjee et al [6] and Luthra et al [18] found no difference in the bioactivity of reconstituted lactate dehydrogenase (LDH) solutions regardless of the state of collapse of the cake.
Stability over Time
Stability over time is perhaps the most important factor for pharmaceutical products due not only to the possible loss of potency but also the possible formation of new, uncharacterized degradation products. Many of the routes of protein degradation are mediated by either the direct participation of water molecules or by the plasticizing effect of water, so it is important to compare the stability of micro or macrocollapsed cakes with the stability of normal cakes having equal moisture contents [7].
In five studies, a broad range of lyophilized protein drug products that included mAb’s and vaccines were subjected to aggressive lyo cycles and exhibited both macro and microcollapse. They were placed on long-term stability at 4 and 25°C with no loss of potency or formation of HMWS species when compared to cakes dried with a conservative lyo cycle [8,15,19-21].
In two other studies, however, some decrease in long term stability was observed. One study found some decrease in the antigenic activity of toxins was observed six months after undergoing an aggressive freeze-drying cycle whether stored at 5° or 25°C [22]. Another study [23] found a decrease in stability of collapsed sucrose-glycine cakes relative to uncollapsed cakes but the collapsed cakes all had elevated moisture levels. A decrease in stability was seen in all cakes with elevated moisture whether made with a conservative or an aggressive cycle.
Conclusions
Exposure of protein drug products to viscous flow resulting in either “macro” or “micro” collapse during primary or secondary drying does not necessarily reduce product quality as long as the residual moisture is reduced to a low enough level for the lyophilized cake to be in the glassy state at the recommended storage condition. Adequate stability of any protein drug product, of course, must be demonstrated on a case by case basis.
References
- S. Rambhatla, J. Obert, S. Luthra, C Bhugra, M. Pikal. 2005. Cake shrinkage during freeze drying: A combined experimental and theoretical study. Pharm Dev Technol 1:33-40.
- R. Johnson, M. Oldroyd, S. Ahmed, H. Gieseler, L. Lewis. 2010. Use of Manometric Temperature Measurements (MTM) to characterize the freeze-drying behavior of amorphous protein formulations. J Pharm Sci 99:2863-2873.
- W. Wang. 2000. Lyophilization and development of solid protein pharmaceuticals. Int J Pharm 203:1-60.
- A. MacKenzie. 1975. Collpase during freeze drying – Qualitative and quantitative aspects. In: S. Goldblith, L. Rey, W. Rothmayr, eds. Freeze drying and advanced food technology. London: Academic Press.
- R. Johnson, C. Kirchhoff, H. Gaud. 2002. Mannitol-sucrose mixtures – Versatile formulations for protein lyophilization. J Pharm Sci 91:914-922.
- K. Chatterjee, E. Shalaev, R. Suryanarayanan. 2005. Partially crystalline systems in lyophilization: II. Withstanding collapse at high primary drying temperatures and impact on protein activity recovery. J Pharm Sci 94:809-820.
- K. Schersch, O. Betz, P. Garidel, S. Muehlau, S. Bassarab, G. Winter. 2010. Systematic investigation of the effect lyophilizate collapse on pharmaceutically relevant proteins I: Stability after freeze-drying. J Pharm Sci 99:2256-2278.
- J. Colandene, L. Maldonado, A. Creagh, J. Vrettos, K. Goad, T. Spitznagel. 2007. Lyophilization cycle development for a high-concentration monoclonal antibody formulation lacking a crystalline bulking agent. J Pharm Sci 96:1598-1608. 9
- E. Meister, H. Gieseler. 2009. Freeze-dry microscopy of protein/sugar mixtures: Drying behavior, interpretation of collapse temperatures and a comparison to corresponding glass transition data. J Pharm Sci 98:3072-3087
- N. Milton, M. Pikal, M. Roy, S. Nail. 1997. Evaluation of manometric temperature measurement as a method of monitoring product temperature during lyophilization. J Parenteral Sci Technol 51:7-15.
- D. Overcashier, T. Patapoff, C. Hsu. 1999. Lyophilization of protein formulations in vials: Investigation of the relationship between resistance to vapor flow during primary drying and small-scale product collapse. J Pharm Sci 88:688-695.
- F. Fonseca, S. Passot, O. Cunin, M. Marin. 2004. Collapse temperature of freeze-dried Lactobacillus bulgaricus suspensions and protective media. Biotechnol Prog 20:229-238.
- A. Abdul-Fattah, V. Truong-Le, L. Yee, L. Nguyen, D. Kolonia, M. Cicerone, M. Pikal. 2007. Drying-induced variations in physico-chemical properties of amorphous pharmaceuticals and their impact on stability (I): Stability of a monoclonal antibody. J Pharm Sci 96:1983-2008.
A. Abdul-Fattah, V. Truong-Le, L. Yee, E. Pan, Y. Ao, D. Kolonia, M. Cicerone, M. Pikal. 2007. Drying-induced variations in physico-chemical properties of amorphous pharmaceuticals and their impact on stability (I): Stability of a vaccine. Pharm Res 24:715-727.
A. Abdul-Fattah, D. Kolonia, M. Pikal. 2007. The challenge of drying method selection for protein pharmaceuticals: Product quality implications. J Pharm Sci 96:1886-1916.. - A. Parker, S. Rigby-Singleton, M. Perkins, D. Bates, D. LeRoux, C. Roberts, C. Madden-Smith, L. Lewis, D. Teagarden, R. Johnson, S. Ahmed. 2010. Determination of the influence of primary drying rates on the microscale structural attributes and physicochemical properties of protein containing lyophilized products. J Pharm Sci 99:4616-4629.
- S. Tchessalov. 2011. Exploring the collapse phenomenon during lyophilization: When is collapse acceptable? CHI PepTalk Conference on Lyophilization and Spray Drying, San Diego.
- L. Lewis, R. Johnson, M. Oldroyd, S. Ahmed, L. Joseph, I. Saracovan, S. Sinha. 2010. Characterizing the freeze-drying behavior of model protein formulations. AAPS PharmSci Tech 11:1580-1590
- A. Dong, S. Prestrelski, S. Allison, J. Carpenter. 1995. Infrared spectroscopic studies of lyophilization- and temperature-induced protein aggregation. J Pharm Sci 84:415-424
- S. Luthra, J. Obert, D. Kalonia, M. Pikal, 2007. Investigation of drying stresses on proteins during lyophilization : Differentiation between primary and secondary-drying stresses on lactate dehydrogenase using a humidity controlled mini freeze-dryer. J Pharm Sci 96:61-70
- S. Bassarab, O. Betz, P. Garidel, S. Muhlau, K. Schersch, G. Winter. 2007. Scrutinizing the effect of collapse during lyophilization. AAPS Annual Meeting Abstract
- S. Jiang, S. Nail. 1998. Effect of process conditions on recovery of protein activity after freezing and freeze-drying. Eur J Pharm Biopharm 45:249-257.
- D. Wang, J. Hey, S. Nail. 2004. Effect of collapse on the stability of freeze-dried recombinant Factor VIII and α-amylase. J Pharm Sci 93:1253-1263.
- S. Passot, F. Fonseca, N. Barbouche, M. Marin. 2007. Effect of product temperature during primary drying on the long-term stability of lyophilized proteins. Pharm Dev Tech 12:543-553.
- B. Lueckel, B. Helk, D. Bodmer, H. Leuenberger. 1998. Effects of formulation and process variables on the aggregation of freeze-dried interluekin-6 (IL-6) after lyophilization and on storage. Pharm Dev Technol 3:337-346.
Author Biographies
Robert Johnson, Ph.D. is a Principal Scientist at the Pfizer Corporation (formerly Pharmacia, or Searle). He earned his B.S. degree in Chemistry (1967) from the University of California at Davis and his Ph.D. (with honors) in 1975 in Biochemistry from Johns Hopkins University School of Medicine. He has been involved in the development of various small molecule and protein drug candidates during his twenty years of industrial experience. In recent years, he has been primarily concerned with developing amorphous and crystalline lyophilized formulations for both high and low concentrations.
Lavinia Lewis, Ph.D. is a Senior Principal Scientist at the Pfizer Corporation (formerly Pharmacia). She earned her Bachelors Degree in Pharmaceutical Sciences in 1993 from the University and Department of Chemical Technology, India; her Masters in Pharmaceutics (1995) from Duquesne University, and her PhD in Pharmaceutical Sciences from the University of Wisconsin at Madison (2001). She has been involved in the development of various lyophilized products for biologics including high concentration formulations, formulations of pegylated protein, vaccines and protein-lipid complexes during her nine plus years of industrial experience.
This article was printed in the April 2011 issue of American Pharmaceutical Review - Volume 14, Issue 3. Copyright rests with the publisher. For more information about American Pharmaceutical Review and to read similar articles, visit www.americanpharmaceuticalreview.com and subscribe for free.