Introduction
During the past 20 years, the field of alternative and Rapid Microbiological Methods (RMMs) has been gaining momentum as an area of research and application across a number of technology sectors. Recent advances in rapid technologies have also encouraged the pharmaceutical and biopharmaceutical industries to validate and implement RMMs in place of their traditional microbiology methods within QC/QA laboratories and on the manufacturing floor.
Many rapid microbiological method technologies provide more sensitive, accurate, precise, and reproducible test results when compared with conventional, growth-based methods. Furthermore, they may be fully automated, offer increased sample throughput, operate in a continuous data-collecting mode, provide significantly reduced time-to-result (e.g., from days or weeks to hours or minutes), and for some RMM platforms, obtain results in real-time. These methods have also been shown to detect slow-growers and/or viable but non-cultural microorganisms as compared with standard methods used today. Most importantly, a firm that implements a RMM in support of sterile or non-sterile manufacturing processes may realize significant operational efficiencies during the monitoring and controlling of critical process parameters, reducing or eliminating process variability, and reducing the risk to patients. Additional benefits may include the elimination of off-line assays and a reduction in laboratory overhead and headcount, lower inventories (raw material, in-process material, and finished product), a reduction in warehousing space, and a decrease in repeat testing, deviations, out-of-specification investigations, reprocessing or lot rejection.
Current rapid method technologies can detect the presence of diverse types of microorganisms or a specific microbial species, enumerate the number of microorganisms present in a sample, and can identify microbial cultures to the genus, species and sub- species levels. The manner in which microorganisms are detected, quantified or identified will be dependent on the specific technology and instrumentation employed. For example, growth-based technologies rely on the measurement of physiological markers that reflect the growth of microorganisms. In fact, these types of RMMs require the organisms from the test sample to proliferate in order to be detected and/or quantified.
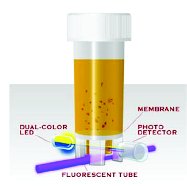
Figure 1 - Test vial confi guration showing the upper incubation zone and the lower reading zone.
Rapid methods that employ the use of growth-based platforms are, for the most part, decreasing the time at which we can detect actively growing microorganisms. Because many currently used growth- based systems continue to use conventional liquid or agar media, the same types of applications that traditional methods are used for can also be applied to growth-based RMMs. Examples include bioburden testing, Microbial Limits, environmental monitoring, sterility testing, and the identification or presence/absence of microorganisms. Examples of the types of core technology principles that are currently used in growth-based RMMs are based on impedance microbiology, the detection of carbon dioxide (CO2), the utilization of biochemical and carbohydrate substrates, the use of digital imaging and auto-fluorescence for the rapid detection and counting of micro-colonies, fluorescent staining and enumeration of micro-colonies by laser excitation, and the use of selective media for the rapid detection of specific microorganisms (see http://rapidmicromethods.com for an online tutorial describing each of these technology platforms). RMM detected by changes in color or fluorescence. Test vials employ a novel dual-zone configuration, where the sample is added to the upper, incubation zone, and an optical sensor monitors changes in color and fluorescence within the lower, reading zone (Figure 1). The upper and lower zones are physically separated from each other, thereby eliminating masking of the optical pathway by sample components or by turbidity arising from dividing microorganisms. Two types of test vials are available:
Currently available growth-based RMMs provide either a quantitative cell count, estimation of viable cell concentration, information regarding the presence of a specific microorganism, or a microbial identification. However, the ability of these RMM systems are limited in scope in that they cannot be used to perform multiple assays using a single technology platform.
A relatively new RMM technology has been introduced that is capable of simultaneously detecting microbial growth, providing an estimation of viable cell counts, and identifying the presence of specific microorganisms, using a novel and automated growth-based format. Case studies demonstrate the ability of the technology to detect total aerobic microorganisms, total yeast and molds, and the presence of E. coli, Staphylococcus, Pseudomonas, Salmonella, as well as additional microorganisms. The system’s scientific principles, case study data and applications will be the focus of the remainder of this article.
Technology Overview
The system is based on monitoring changes in a broth medium with unique dyes in which target microorganisms grow and are the membrane vial for the detection of specific types of microorganisms, and the universal carbon dioxide (CO 2) sensor vial for estimating the concentration of viable organisms. Both of these vial types can be simultaneously used for the automated detection of microorganisms in the same instrumentation.
The membrane vial format contains a specific growth medium and indicator dyes that are identical in both the incubation and detection zones. Prior to a sample being introduced, the color or fluorescence observed in the detection zone is identical to the corresponding color or fluorescence in the incubation zone. After the sample is introduced into the incubation zone, if microorganisms are present and they can grow in the specific medium, the subsequent changes in color or fluorescence will be monitored in the detection zone. As previously discussed, turbidity as a result of microbial growth is retained in the incubation zone because microorganisms will not pass through the membrane separating the upper and lower sections of the vial.
The universal CO2 sensor vial contains a solid sensor that changes color when CO2 diffuses into the sensor located in the bottom of the vial. Only gases can penetrate the sensor, while the media, microorganisms and particulate matter remain in the upper, inoculation zone. Microorganisms, when grown in liquid culture, produce CO2 and other metabolites. In the closed CO2 sensor vial, when CO2 from the medium diffuses into the dark-colored sensor, the sensor changes to a yellow color, which is easily detected by the system.
Both of these types of vials are used for the detection of specific organisms or for the estimation of the number of organisms in the original sample. For example, the Total Aerobic Count Vial and the Total Yeast and Mold Vial utilize the CO2 sensor platform, where a general medium is used in the Total Aerobic Count Vial, and a selective medium with added antibiotic (to suppress the growth of bacteria) is used in the Yeast and Mold Vial. The Enterobacteriaceae Vial monitors a change in color due to a pH shift as Enterobacteriaceae organisms (Gram negative, bile tolerant) ferment glucose in the presence of a selective medium. The Coliform Vial monitors a change in color due to a pH shift as coliform bacteria ferment lactose in the presence of a different selective medium. The E. coli Vial monitors the utilization of MUG (4-Methylumbelliferyl-3-D-Glucuronide). The Staphylococcus Vial monitors changes in color due to a pH shift as Staphylococcus utilizes mannitol in another selective medium. Both the Pseudomonas and Salmonella Vials use the same CO2 sensor platform as in the Total Aerobic Count Vial, but each utilize a specific selective medium for the growth of each organism.
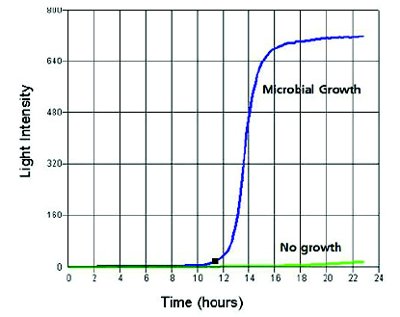
Figure 2 - Response to microbial growth. Light intensity in the reading zone of the test vial will increase when microbial growth occurs, and as the threshold detection level is reached (blue line). If there is no microbial growth, the threshold detection level is not reached (green line).
The sensitivity of the system is a single viable cell per sample vial; when a single cell replicates to a specific detection threshold level, a positive response is recorded, and the time to result (in hours) is provided. The time to result is based on the threshold level for detection, which is ~100,000 cells/ml for bacteria, and ~10,000 cells/ml for yeast and mold. An example of the system’s response to microbial growth is illustrated in Figure 2. Additionally, the system typically yields faster results than the conventional plate count method; one bacterial cell is usually detected within 8-18 hours, a single yeast cell is detected in 20-30 hours, and mold requires 35-48 hours for detection.
The instrument, which is temperature-controlled and operates within a range of 15-60°C, collects the optical data generated from each vial at a rate of 10 readings per hour.
Estimating the Number of Viable Microorganisms in the Test Sample: The Dilute-to-Specification Method
In addition to detecting the presence of specific microorganisms, each of the test vials may be used to estimate the number of organisms in the original test sample by first diluting the sample to the specification limit required for in-process or finished product release. The diluted sample is then introduced into the relevant test vial. If there is microbial growth in the vial, the number of organisms in the original sample is higher than the required specification level; if there is no growth in the vial, then the number of organisms in the original sample is lower than the required specification level. Although this is a semi-quantitative method, this procedure is appropriate for determining if a test sample is above or below a threshold level that is directly correlated with a quantitative specification, action or alert level. This is similar to using liquid medium in a Most Probable Number, or MPN procedure. A related method has previously been utilized for the release of non-sterile pharmaceuticals using an ATP bioluminescence RMM.
For example, let’s assume that a sample under test has a specification of less than 100 CFU/mL. If the sample is first diluted 1:100, and there were 500 CFU/mL in the original sample, the 5 CFU would be introduced into the vial’s growth medium when 1 mL of the dilution is added to the test vial. These 5 CFU would replicate, and the system would detect microbial growth, indicating that the sample contained greater than the acceptable specification level. Conversely, if there were 10 CFU/mL in the original sample, a 1:100 dilution would result in the addition of less than 1 CFU (theoretically 0.1 CFU) when 1 mL of the dilution is added to the test vial. In this case, there should be no growth detected, and the sample would meet the specification of less than 100 CFU/mL.
Absence of Specified Organisms
For test samples that have requirements for the absence of specified organisms, a pre- incubation step can be incorporated into the procedure described above. For example, the sample can be prepared using a 1:10 dilution of not less than 1 g of the sample in an appropriate growth medium. Following an incubation period, 1 mL of the 1:10 dilution can then be transferred to the Test Vial specific for the specified organism to be detected.
Case Studies in Validation and Comparative Testing
Method validation is the process used to confirm that an analytical procedure employed for a specific test is reliable, reproducible and suitable for its intended purpose. All analytical methods need to be validated prior to their introduction into routine use, and this is especially true for novel technology platforms, such as RMMs. The current technology has been put through its paces with regard to method validation, and I will share a few of the results in this article. Particularly, we will review validation data from Specificity, Limit of Detection (LOD) and Precision testing, as well as comparative analyses for the estimation of cell counts.
Specificity Testing
Specificity is the ability to detect a range of microorganisms, which is one of the validation expectations required to demonstrate that this method is fit for its intended purpose. When the method is intended to detect a single type of microorganism, or a specific class of microorganisms, it is necessary to demonstrate that the target organisms are, in fact, detected, while other non-target organisms are not detected. This is also referred to as inclusivity and exclusivity
Table 1 - Results from specificity testing.

For each of the test vials previously described, target organisms that should be detected within the test vial were inoculated at levels ranging from 10 to 1,000 CFU/vial, and non-target organisms were inoculated at levels ranging from 10,000 to 100,000 CFU/vial. Results are shown in Table 1.Briefl y, 125 diverse species of bacteria were separately inoculated into the Total Aerobic Count Vial. All of the organisms tested were detected in the vials (no non-target testing was conducted, as the growth medium in this vial is universal). Next, 42 diff erent yeast and molds were evaluated in the Yeast and Mold Vial. All of the yeast and mold were detected. However, when 25 species of various bacteria were evaluated in the Yeast and Mold Vial, none of the organisms were detected. When 52 diff erent bacteria belonging to the Enterobacteriaceae family were inoculated into the Enterobacteriaceae Vial, all of these organisms were detected; however, when 23 Gram- negative and 28 Gram-positive species of bacteria that did not belong to the family Enterobacteriaceae were evaluated, none of these non- target organisms were detected (see Table 1). Similar results were obtained for the E. coli, Staphylococcus, Pseudomonas and Salmonella Vials (data not shown). Therefore, inclusivity for the target organisms and exclusivity for the non-target organisms was demonstrated.
Table 2 - Results from limit of detection testing. ND= no growth detected in the RMM.
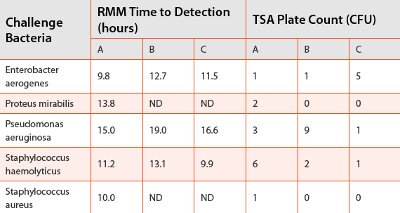
Limit of Detection Testing
Limit of Detection (LOD) is the lowest number of microorganisms in a sample that can be detected under the stated experimental conditions. The LOD was first evaluated by inoculating 1 mL of a variety of standardized bacterial species, with a target level of between 1-5 CFU/mL, in triplicate Total Aerobic Count Vials and on triplicate Tryptic Soy Agar (TSA) plates. The ability of the RMM to detect the presence of these low levels of bacteria in the Total Aerobic Count Vials was compared with the presence of CFUs on the TSA plates. Detection in the RMM was expressed as the number of hours required for the system to confirm microbial growth. A summary of the results for some of the challenge organisms is presented in Table 2 (where A, B and C correspond to the triplicate samples).
Table 3 - Results of E. coli testing in the presence of neutralized product. ND = no growth detected in the RMM.
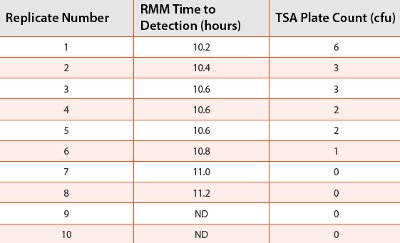
The results from these studies indicate that the RMM is capable of detecting very low levels of challenge bacteria when the same inoculated organisms are recovered on TSA plates. Additionally, the RMM did not detect growth when the same inoculum gave 0 CFU on the TSA replicate plates. A similar study using C. albicans and A. brasiliensis (niger) inoculated into Total Yeast and Mold Vials and on Sabouraud Dextrose Agar (SDA) plates, and with Citrobacter freundii inoculated into Enterobacteriaceae Vials and on Violet Red Bile Glucose (VRBG) plates, gave comparable results (data not shown).
In a separate experiment, a suspension of E. coli at a concentration of 120 CFU/mL was inoculated into a hair styling gel product at a dilution of 1:10. Aliquots of the inoculated product were then added to TSB with neutralizers in another 1:10 dilution. One (1) mL of the neutralized product was separately dispensed into ten (10) replicateTotal Aerobic Count Vials and on ten (10) replicate TSA plates. The resulting concentration of E. coli added to each test vial and on each TSA plate would have been 1.2 CFU. The ability of the RMM to detect thepresence of E. coli in the test vials was compared with the presence of CFUs on the TSA plates. A summary of the results is presented in Table 3.
Similarly as in the previous study, the results from this E. coli testing indicate that the RMM is capable of detecting very low levels of challenge bacteria from neutralized product when the same inoculated organisms are recovered on TSA plates. Furthermore, the RMM did not detect growth when the same inoculum gave 0 CFU on the TSA replicate plates. However, there were two instances where the RMM detected growth while there were no CFUs on the agar plates, suggesting that in some instances, the RMM is more sensitive at detecting low levels of microorganisms as compared with the traditional agar plate method.
Table 4 - Average %CV, based on detection times.
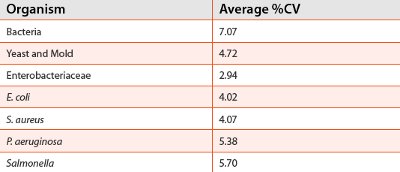
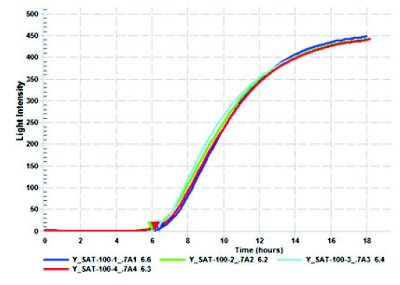
Figure 3 - Example of replicate detection plots for S. aureus.
Precision Testing
Precision is the degree of agreement among individual test results when the procedure is applied repeatedly to multiple samplings of suspensions of microorganisms across the range of the test. Precision is usually expressed as the variance, standard deviation or coeffi cient of variation (CV) of a series of measurements. Generally, a %CV of 15-35% is acceptable. For the purpose of demonstrating precision in this RMM, a series of replicate tests were performed using diff erent concentration levels and a variety of challenge microorganisms, including seven (7) bacterial species in the Total Aerobic Count Vials, seven (7) yeast and eight (8) mold species in the Total Yeast and Mold Vials, 23 strains of Enterobacteriaceae in the Enterobacteriaceae Vial, and two (2) diff erent strains of E. coli, S. aureus,Salmonella and Pseudomonas aeruginosa, which were each assessed in their respective test vials. The average %CV for the challenge organisms is presented in Table 4. To illustrate precision in microbial detection for a representative microorganism, Figure 3 illustrates a graph demonstrating precision for a representative test organism, S. aureus.
The data from these studies demonstrate that the degree of agreement among individual test results, when the rapid method is applied repeatedly to multiple samplings of suspensions of more than 50 diff erent species and strains of a wide variety of microorganisms, is well within the acceptable range for a suitable level of precision.
Comparative Testing of Standardized Cultures According to the RMM and USP<61>and USP<62>
USP<61>and USP<62> describe the tests for the microbiological examination of nonsterile products with respect to the enumeration and presence of specified microorganisms. A series of studies were conducted to determine if the RMM is comparable with these pharmacopeial procedures. The first set of experiments involved the use of pure cultures of microorganisms to determine if the RMM would detect a range of total aerobic counts similarly as the USP<61> method. Cultures were first diluted to provide a range of challenge suspensions. The resulting suspensions were then used to represent nonsterile product that would either be above or below a certain USP specification level for total aerobic count. In order to compare the RMM’s ability to detect organisms that were above or below these specification levels, a dilute-to-spec method was used on the challenge suspensions as described earlier in this manuscript. For example, if we want to evaluate whether the RMM would detect microorganisms that were either less than or greater than a specification level of 100 CFU/mL, we would make a 1:100 dilution of the selected challenge suspension, and add this to the Total Aerobic Count Vial. Detection of growth would indicate that the original suspension had organisms in excess of 100 CFU. Conversely, if no growth is detected, then the original suspension would have had less than 100 CFU. The standard plate count method was used on the same suspension to represent the USP method. Fifty-nine (59) tests were conducted with B. subtilis, E. coli, P. aeruginosa and S. aureus using various suspension concentrations in the range of 10-100,000 CFU/ml, and evaluated for being either above or below the following specification levels: 101, 102, 103, 104, and 105. There was 100% agreement between the RMM and the USP<61>methods in determining that the number of organisms found in each sample were either above or below the specification level.
Similar results were obtained when 33 tests were conducted with A. brasiliensis, C. albicans and S. cerevisiae at various concentrations in the range of 10-1,000 CFU/ml and evaluated in the Total Yeast and Mold Vial, and when 44 tests were conducted with Enterobacteriaceae at various concentrations in the range of 10- 1,000 CFU/ml.
To assess whether the RMM would detect the presence of low levels of specified organisms, suspensions containing between 1-5 CFU of multiple strains of E. coli, S. aureus, P. aeruginosa and Salmonella were separately evaluated in their respective test vials and the results were compared with testing the same suspensions for the presence of specified organisms according to USP<62>. Additionally, organisms that would not expect to be detected by either method (exclusivity) were also evaluated in each test vial and in each USP<62> assay (e.g., S. aureus was used in the test for E. coli in both the RMM and the USP methods). There was 100% agreement between the RMM and the USP<62> methods with respect to the detection of the correct specified organisms (inclusivity). There was also 100% agreement between the two methods when organisms should not have been detected (exclusivity).
Table 5 - Testing for Total Count in OTC Products.
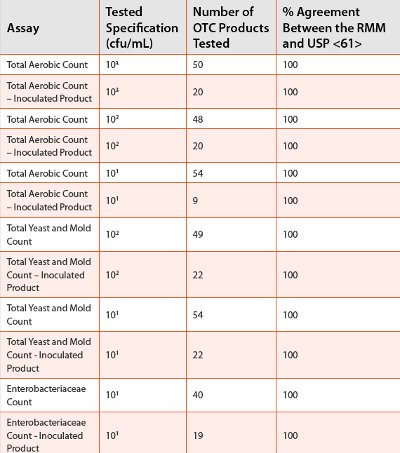
Comparative Testing of Nonsterile Products According to the RMM and USP<61>and USP<62>
Over-the-counter (OTC) products that were known to contain certain levels of microorganisms were tested for meeting a variety of specification levels for microbial counts as well as for the presence of specified organisms as described in the previous section and for both methods. Additionally, many of the OTC products were inoculated to ensure that the number of organisms would be present in the products at a concentration of at least the target specification level. A summary of the results is presented in Tables 5 and 6.
Table 6 - Testing for the Presence of Specified Organisms in OTC Products.
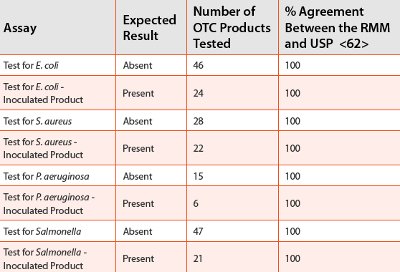
The results from these studies clearly indicate that the RMM is capable of determining whether microorganisms are present in amounts that either exceed or are below a predetermined specifi cation level, and can detect the presence of specified organisms in a wide variety of OTC products.
Summary
The results from all of the testing between the RMM and the conventional or pharmacopoeial methods are comparable.
Furthermore, the system demonstrates excellent specifi city (inclusivity and exclusivity), precision and the ability to detect levels of microorganisms that are equivalent or better than the conventional plate count method.
The system is able to adapt to many diff erent microbiological applications, including, but not limited to, raw material assessments, in-process analysis and fi nished product testing, but especially for the compendial testing of nonsterile products.
Finally, the ability of this novel RMM technology to provide both an estimation of cell count as well as testing for the presence or absence of specified microorganisms is a benefi t not available with most other rapid methods that are commercially available. The system has already been successfully used within the food, beverage, cosmetic, nutraceutical and dietary supplement sectors, and is now positioned to support the needs of the pharmaceutical industry as well.
Reference
- 2011. Miller, M.J., Microbiology Consultants, LLC, and Eden, R., BioLumix. Case Study of a New Growth-Based Rapid Microbiological Method (RMM) That Detects the Presence of Specific Organisms and Provides an Estimation of Viable Cell Count. PDA 6th Annual Global Conference on Pharmaceutical Microbiology. Washington, DC.
Author Biography
Dr. Michael J. Miller is an internationally recognized microbiologist and subject matter expert in pharmaceutical microbiology and the design, validation and implementation of rapid microbiological methods. He is currently the President of Microbiology Consultants, LLC (http://microbiologyconsultants.com). For more than 24 years, he has held numerous R&D, manufacturing, quality, and consulting and business development leadership roles at Johnson & Johnson, Eli Lilly and Company, Bausch & Lomb, and Pharmaceutical Systems, Inc. In his current role, Dr. Miller consults with multinational companies in providing technical, quality and regulatory solutions in support of RMMs, sterile and non-sterile pharmaceutical manufacturing, contamination control, isolator technology, validation and microbiological PAT. He also provides comprehensive training for his clients in the areas of rapid method validation and implementation.
Dr. Miller has authored over 100 technical publications and presentations in the areas of rapid microbiological methods, PAT, ophthalmics, disinfection and sterilization, is the editor of PDA’s Encyclopedia of Rapid Microbiological Methods, and is the owner of http://rapidmicromethods.com, a website dedicated to the advancement of rapid methods. He currently serves on a number of PDA’s program and publication committees and advisory boards, is co-chairing the revision of PDA Technical Report #33: Evaluation, Validation and Implementation of New Microbiological Testing Methods, and routinely provides RMM training programs for the industry and professional organizations worldwide.
Dr. Miller holds a Ph.D. in Microbiology and Biochemistry from Georgia State University (GSU), a B.A. in Anthropology and Sociology from Hobart College, and is currently an adjunct professor at GSU. He was appointed the John Henry Hobart Fellow in Residence for Ethics and Social Justice, awarded PDA’s Distinguished Service Award and was named Microbiologist of the Year by the Institute of Validation Technology (IVT).