Biology and Horticulture Department
Biology and Horticulture Department
Biology and Horticulture Department
Biology and Horticulture Department
Abstract
Escherichia coli is one of the specific bacterial indicators required by the United States Pharmacopeia which can be considered hazardous to consumers if found in non-sterile pharmaceutical products. Standard microbiological testing requires four to five days to be completed to show the absence of E. coli from raw materials and finished products. A Real-Time (RT) PCR assay was developed to detect E. coli in pharmaceutical products contaminated with low levels of bacterial contamination. Different pharmaceutical suspensions were artificially contaminated with Burkholderia cepacia, Escherichia coli, Staphylococcus aureus, and Bacillus megaterium. After a 24-hour incubation in TSB with Tween 20, bacterial DNA was extracted from each sample by using a Tris-EDTA, proteinase K, Tween 20 buffer. Regular PCR targeting the 1.5 kilobases 16S rRNA eubacterial gene and cloning showed the predominant DNA in the extracted mix belonged to E. coli. Selective media isolation of bacterial contamination showed E. coli detected on MacConkey or eosine methylene blue agar. RT-PCR using primers UAL754 and UAR900 amplified a 147base pair betaglucuronidase DNA fragment using a LightCycler system with SYBR green I, a common double-stranded binding dye. The cycle at which fluorescence from amplification exceeds the background fluorescence was referred as quantification cycle (Cq). All samples were found to be positive by standard microbiological testing and RT-PCR. E. coli was detected within 30 hours in all contaminated samples using RTPCR. Based upon standard curve analysis of E. coli DNA, the minimum DNA concentration that could be detected was 0.00109 ng/μl with a correlation value of 0.96.
Introduction
Pharmaceutical products are susceptible to microbial contamination when companies do not follow proper processes to provide safe, efficacious, and stable products.1 Pharmaceutical processes are highly technical and detailed operations, which require specialized personnel and complex procedures to control the continuity, reproducibility, and stability of the different systems put in place to guarantee the compliance with good manufacturing processes (GMP). Because microorganisms are ubiquitous to the environment, during construction of pharmaceutical facilities, systems are developed and validated to contain and control their numbers, distribution, and growth. Pathogenic microorganisms cause morbidity and in some cases mortality when present in pharmaceutical products.2,3
Product spoilage due to microbial breakdown of formulations can also affect potency and shelf life. Manufacturing non-sterile pharmaceuticals is very challenging because processes and products contain a microbial bioburden that is not detrimental to the formulation and hazardous to consumers. The challenge to pharmaceutical manufacturers is to produce a non-sterile drug with low levels of microorganisms and without the wrong type of objectionable microbes. Some guidance to determine what is objectionable is provided by the Code of Federal Regulations and the USP informational chapter <1111>.3
Based upon compendial tests, when non-sterile pharmaceuticals are tested, objectionable microorganisms must be absent from the finished product and in some cases also from raw materials.2,3 For this purpose, regulatory agencies around the world require the absence of certain types of microorganisms that can be considered hazardous to consumers or compromise drug stability and efficacy.2Escherichia coli is one of the indicator microorganisms that must be absent from non-sterile pharmaceutical drugs. Furthermore, based upon published scientific studies, E. coli is a frequent microbial contaminant in nonsterile pharmaceutical products around the world.3,4 The presence of E. coli in non-sterile or any type of microorganism in sterile products indicates lack of process control and system optimization.3
E. coli is also one of the U.S. Environmental Protection Agency (EPA) recommended indicator organisms for freshwater systems and is a sensitive measure of fecal pollution since it is common to almost all warmblooded animals, including humans.5 Most types of E. coli are harmless but some strains can cause gastrointestinal disorders if you eat contaminated food or drink fouled water. E. coli is also responsible for pneumonia, wound infections, sepsis, and urinary tract infections (UTIs) in hospitals.6 Around 75% to 95% of UTIs are caused by infections with E. coli. Furthermore, E. coli is the most common gram-negative bacterial etiological agent in nosocomial infections in the United States.7
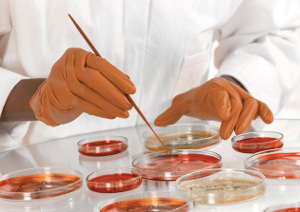
Most pharmaceutical companies relied on traditional cultivation and phenotypic methods to isolate and identify microbial contaminants.8 These methods are time consuming (five to seven days) and laborious. They relied upon the growth of microorganisms on the specific substrates in the media. However, some bacteria do not grow on those substrates or grow extremely slow to be detected by the incubation times currently used. If the processes and systems are not validated or properly implemented, microorganisms can contaminate products, raw materials, and equipment.1-3 However, microbial contamination is not always caused by pure cultures.2,3 Several non-sterile pharmaceutical products recalled by the FDA did not specify the type of microbial contamination indicating that different types of microorganisms were present in the products.2,3 Of 134 non-sterile products recalled, 20% were described as microbial contamination, bacterial contamination, or exceeds microbial limits.2 Unfortunately, there was no description of the genus or species causing the contamination event. Microbial identification could have provided valuable information to determine the contamination source.
Rapid PCR detection of E. coli contamination in pharmaceutical samples has been previously reported.9-12 Rapid PCR detection showed optimization of quality control testing leading to greater process control during manufacturing of non-sterile pharmaceuticals. Different pharmaceutical formulations were contaminated with pure cultures of E. coli or mixed bacterial cultures with levels of contamination ranging from 10 to 1000 colony forming units (CFU). Detection of amplified fragments was performed using gel electrophoresis. However, there are no scientific studies on the application of Real-Time (RT)-PCR to detect E. coli in different pharmaceutical formulations. RT-PCR detects and quantifies the target DNA without the need to perform any other analysis.
Subscribe to our e-Newsletters
Stay up to date with the latest news, articles, and events. Plus, get special offers
from American Pharmaceutical Review – all delivered right to your inbox! Sign up now!
The objective of this study was to develop a RT-PCR protocol for the detection of E. coli in different non-sterile pharmaceutical delivery systems such as tablets, creams, and liquid products containing low levels of bacterial contamination with different bacterial species.
Materials and Methods
Samples. Different types of pharmaceutical formulations were analyzed. They ranged from liquid, creams, and tablet formulations. The products were purchased at a local pharmacy store located in Fair Lawn, N.J., U.S.A.
Microorganisms. The microorganisms spiked into product suspensions were: Burkholderia cepacia ATCC 25416, E. coli, Bacillus megaterium, and Staphylococcus aureus. All microbial identifications were confirmed by16S rRNA sequencing.
Inoculum preparation. Bacterial cultures of S. aureus, B. megaterium, and E. coli were shaken, 200 rpm, for 24 hours at 37°C. B. cepacia cultures were shaken for 48 hours. After incubation, all bacteria were diluted with sterile water to obtain a final inoculum ranging from 10 to 100 CFU/ml.
Sample inoculation and incubation. One gram of each pharmaceutical product was added to 100 ml of trypticase soy broth (TSB) containing 2% or 4% Tween 20. After product addition, samples were inoculated with serial dilutions of all four bacteria followed by incubation at 37°C for 24 hours with shaking, 200 rpm.
Microbiological analysis of samples. After incubation in TSB, samples were streaked onto the following selective agar media: eosin methylene blue (EMB), and MacConkey (MAC) agar. All selective media plates were incubated for 48 hours at 37°C. Random colonies grown on selective media plates were identified by gram stain, biochemical, and genetic analysis.
DNA extraction. Bacterial DNA was extracted from contaminated product suspensions and colonies using a Tris-EDTA buffer containing Tween 20 and proteinase K.9,10 Suspensions were incubated for 20 minutes at 37°C followed by ten minutes at 95°C. Different aliquots of extracted bacterial DNA were used in the PCR reactions.
E. coli DNA was extracted from pure cultures as previously described.13 Fluorometric quantitation of E. coli DNA concentration was determined using a procedure previously described.13 After quantitation, ten-fold serial dilutions of E. coli DNA were used as standard curves. Negative controls based upon sterile water were included in RT and regular PCR assays.
Regular PCR. Bacterial DNA extracted from contaminated product suspensions was amplified using PCR beads.9 The amplification reactions targeted the 1.5 kilobases (kb) 16S rRNA eubacterial gene.13 Amplicon detection was carried out by gel electrophoresis using DNA Cassettes containing 1.2% agarose.13 A DNA Marker with fragment sizes ranging from 100 base pair (bp) to 4 kb was used to determine the presence of correct DNA gene fragments.
Cloning libraries of eubacterial 16S rRNA genes. The DNA fragments from the PCR amplification of eubacteria 16S rRNA genes were cloned using plasmid pCR®4-TOPO according to the manufacturer’s instructions. Transformations were performed as previously described.13 White colonies grown on Luria Bertani (LB) Agar with ampicillin (50 μg/ml) were transferred to LB broth containing ampicillin (50 ug/ml). Samples were incubated overnight at 37°C.
Plasmids were isolated from each clone using a procedure previously described.13 Cloned inserts were reamplified using M13 DNA primers. DNA sequencing reactions of the amplified PCR fragments were performed as described by Jimenez et al.13 Homology searches for 16S rRNA genes were performed using the Classifier application on the Ribosomal Database Project (http://rdp.cme.msu.edu/classifier/classifier.jsp)14 and also using the GenBank server of the National Center for Biotechnology Information (NCBI) (http://blast.ncbi.nlm.nih.gov/Blast.cgi) and the BLAST algorithm.15
RT-PCR reactions. Bacterial DNA extracted from contaminated product suspensions was used to develop the RT-PCR assay. RT-PCR was performed with a LightCycler System13 using SYBR green PCR master mix as the detection system in a reaction mixture of 25 ul. Aliquots of 3 ul of extracted bacterial DNA from pure cultures and product suspensions were used for all reactions.
DNA primers UAL754 and UAR900 amplify a 147bp fragment of the E. coli beta-glucuronidase (uidA) gene.5 Different reactions conditions and cycles were analyzed to optimize target detection. However, the optimized reaction conditions were a preincubation cycle of 95°C for 600 sec, three step amplification of 35 cycles of 95°C /10 sec, 58°C/10 sec, 72°C/20 sec, and a melting cycle of 95°C /10 sec., 65°C/60 sec, 97°C/15 sec. The cycle at which fluorescence from amplification exceeds the background fluorescence was referred as quantification cycle (Cq).
After RT-PCR amplification, contaminated pharmaceutical samples were analyzed by gel electrophoresis.13 Amplified uidA fragments were sequenced using primer UAL754. Homology searches for the sequenced uidA fragments were performed using the GenBank server of the National Center for Biotechnology Information (NCBI) (http://blast.ncbi.nlm.nih.gov/Blast.cgi) and the BLAST algorithm.15
Results and Discussion
Low levels of B. cepacia, S. aureus, E. coli, and B. megaterium were spiked into non-sterile pharmaceutical formulations, with different delivery systems, to simulate a contamination event caused by four different bacterial species. All bacteria used in the study came from species previously reported to be major contaminants leading to products recalled in the USA.2,3 The spiked inoculum numbers were below 62 CFU/ml (Table 1). In order to accelerate bacterial growth, after inoculation, samples were shaken for 18-24 hours at 37°C. Different concentrations of Tween 20 were added to TSB to determine the optimal concentration to recover bacteria from all the spiked pharmaceutical products. Because of the different chemical composition of the products, concentrations ranging from 0.5% to 3.0% Tween 20 were tested. The optimal concentration was found to be 2%. TSB enrichment media containing 2% Tween 20 inhibited the antimicrobial activity of the formulations allowing rapid bacterial growth.
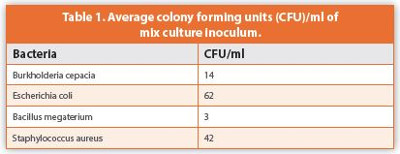
After shaking, samples were streaked onto media that selectively isolated Gram-negative bacteria, e.g., MAC and EMB. Table 2 shows the results for each selective agar media analyzed. All ten products tested were found to show bacterial growth. Identification of colonies growing on selective agar media for gram-negative bacteria showed the presence of E. coli (Table 2). Although B. cepacia is a Gram-negative bacterium and the use of MAC has been previously reported to be used in recent outbreaks,16 results demonstrated that E. coli outcompeted B. cepacia on MAC. However, when Pseudomonas isolation agar (PIA) was used, B. cepacia was easily isolated from all products.13 Both B. cepacia and S. aureus were also detected in all products by selective agar media and specific RT-PCR testing.13,17 Bacterial contamination was confirmed to be mix with the viable presence of all bacterial species.13,17
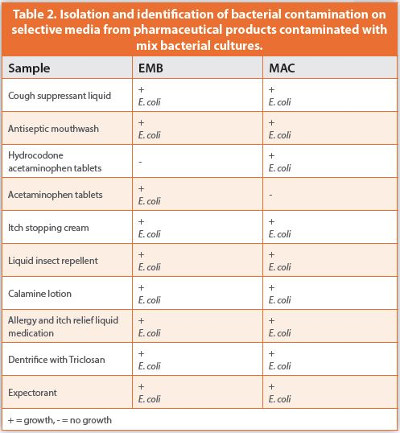
E. coli was isolated from all products on both agar media with the exception of acetaminophen tablets and hydrocodone acetaminophen tablets. For acetaminophen tablets only EMB was able to recover E. coli while effective recovery was found using MAC with hydrocodone acetaminophen tablets (Table 2). Phenotypic and genetic identification identified all colonies to be E. coli.
Contaminated pharmaceutical samples inoculated with the mix bacterial culture were analyzed by a quick DNA extraction procedure using Tris-EDTA buffer, Tween 20, and proteinase K.9,10 The procedure lasted only 30 minutes and did not require organic solvents or hazardous chemicals. To determine the predominant bacterial DNA in the extracted product suspensions, a regular PCR reaction was performed targeting the 1.5 kb 16S rRNA eubacterial gene. The 1.5 kb fragment is a universal gene present in all members of the domain bacteria.18 The gene has conserved and variable regions that can be used to distinguish bacterial phyla, genera, and species. The amplified fragments from product suspensions were cloned into vector plasmid pCR®4-TOPO. DNA sequencing of bacterial clones demonstrated the predominant presence of E. coli in the clone library. No other bacterial 16S rRNA sequence was detected. Evidently, E. coli outcompeted B. megaterium, S. aureus, and B. cepacia in all TSB enrichment samples.
To specifically detect E. coli in the extracted bacterial DNA a 147bp fragment of the uidA gene was used as a target to develop a RT-PCR assay. The E. coli genome size ranged from 4.6 to 5.3 million bases (Mb).19 The sensitivity and accuracy of the primer pair UAL-754 and UAL-900 to detect the uidA gene was previously described.5,9,10,20 The copy number for the uidA gene is 1.21 Standard curves of E. coli DNA were constructed to determine the DNA concentration of E. coli uidA gene in pharmaceutical samples contaminated with B. megaterium, S. aureus, E. coli, and B. cepacia. The DNA concentration for undiluted samples of E. coli DNA was found to have 1.09 nanograms(ng)/microliters (μl) with a Cq of 12.46 (Table 3). When ten-fold dilutions of E. coli DNA were tested, the minimum concentration of uidA gene detected was 0.00109 ng/μl with a correlation coeffi cient of 0.96 (Figure 1). The DNA concentration at that dilution level is equivalent to 10 to 100 cells. The Cq for the lowest DNA concentration detected was 31.62 (Table 3). Melting curve analysis of the amplified DNA confirmed the presence of the 147bp fragment (Figure 2). Furthermore, gel electrophoresis of the different DNA concentrations showed the presence of a 147bp fragment at the lowest detectable concentration (Figure 3, lanes 1-7). Lower sensitivity levels were found in B. cepacia studies when a specific 16S rRNA gene was targeted to detect B. cepacia in pharmaceutical products contaminated with different bacterial species.13 The lower detection level was based upon the fact that the 16S rRNA gene has a higher copy number, e.g., 6, on the B. cepacia genome. However, previous studies using multiplex and regular PCR to detect E. coli in pharmaceutical products targeted a 16S rRNA gene fragment which can provide a lower detection level since there is a higher copy number for that gene, e.g., 6, compared to 1 copy for the uidA gene.11,12
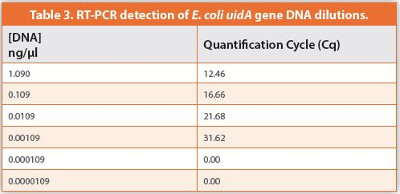
All contaminated products analyzed were found to be positive by the RT-PCR assay. Table 4 shows the Cq values for each pharmaceutical product tested. The Cq indicated the cycle at which fluorescence from amplifycation exceeded the background fluorescence when the target sequence was detected. Negative controls did not show any reaction and were completely devoid of any signal or band as per melting curve and gel electrophoresis analyses (Figures 2 and 4A). The 147bp DNA fragment of the beta-glucuronidase gene was detected in all samples (Figure 3, lanes 8-11; Figure 4A). DNA sequencing and BLAST analysis of the amplified E. coliuidA gene confirmed the identity of the target gene in the contaminated samples (Figure 4B).
Cq values for artificially contaminated pharmaceutical products ranged from 14.06 to 31.07. The average Cq value for all ten formulations was 19.74 with seven showing values lower than the average which demonstrated that E. coli was accurately detected in less than 20 cycles for most samples. This indicated that the cycle time for those seven formulations can be significantly reduced from 35 to maybe 20. Because all pharmaceutical samples were tested simultaneously, the optimal cycling time was found to be 35. However, during routine quality control testing, each product will have their own test method specifications with different cycle times and incubation conditions. Preliminary studies have shown that the reduction of cycling time for some of the products did not affect the Cq values for E. coli. When compared to B. cepacia and S. aureus, E. coli showed faster Cq values in the RT-PCR reaction, the average Cq value for E. coli was shorter than B. cepacia and S. aureus13,17 (Table 5). B. cepacia Cq value was the longest of all three bacteria with an average of 24.82 while S. aureus was 22.14.13,17 The products with the fastest Cq values for B. cepacia and S. aureus were dentifrice with triclosan and hydrocodone acetaminophen tablets, respectively. The longest Cq values for B. cepacia were found with hydrocodone acetaminophen tablets while for S. aureus was acetaminophen tablets (Table 5). The faster Cq values for E. coli were found with the cough suppressant liquid while hydrocodone acetaminophen tablets showed the longest. This indicated that the growth conditions and neutralization protocol provided optimal conditions for the low numbers of E. coli to grow enough in the presence of other bacteria. Therefore, bacterial DNA can be extracted from the bacterial mix culture using a mild DNA extraction protocol and subsequently detected by the RT-PCR. The low E. coli inoculum of 62 CFU/ml was easily detected after a 24 hour enrichment with shaking at 37°C even in the presence of other bacterial species.
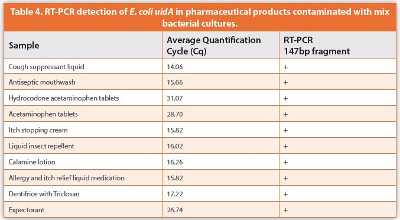
Samples pre-enrichment was a very important step since the low numbers of bacterial contamination must reach the minimum detectable levels for the DNA to be extracted and the target gene to be detected. Enrichment also provided for the dilution of inhibitory substances that could have interfered with bacterial growth and the RT-PCR reaction. Several commercial DNA extraction procedures were tested. Detection of the E. coli uidA fragment was sporadic or negative due to the need for these procedures to have multiple steps of column purifications, centrifugations, and washes. A two-step DNA extraction procedure was optimized to transfer an aliquot of the suspensions into Tris-EDTA buffer containing Tween 20 and proteinase K. The extraction method was efficient to get enough DNA for RT-PCR analysis. Pre-enrichment is usually overlooked in the pharmaceutical industry.8,11 Bacterial cells undergo a physiological state by reducing metabolic reactions rates and cell size which are affecting the protein and enzymatic profiles used to identify environmental isolates.8 These cell changes are triggered by physical processes and environmental systems implemented to reduce or eliminate microorganisms during pharmaceutical manufacturing. The cells are injured and need time and conditions to recover and grow. This was previously demonstrated when Salmonella typhimurium was not recovered on growth media nor detected by PCR testing from pharmaceutical samples in the presence of mix bacterial cultures unless a new enrichment broth, buffered peptone water, was used.22
RT-PCR detection of E. coli in contaminated products can provide a rapid quality control analysis of compromised products preventing any major outbreak due to the use of contaminated material by consumers and healthcare providers.1,2 Standard methods required from five to six days to complete the isolation and identification of E. coli from contaminated samples. However, the RT procedure developed in this study was completed in less than 30 hours. Bacterial DNA extraction from product suspensions was completed in less than 35 minutes. The RT-PCR set up and the thermocycler run were completed within two hours. Data analysis did not take more than five minutes. Since the assay is detecting the amplified product in real time, the instrument screen provides on-going real time data analysis where the amplification curves can be used to predict a presumptive positive result before the assay is completed.
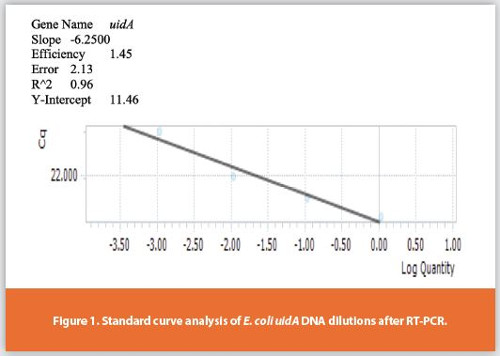
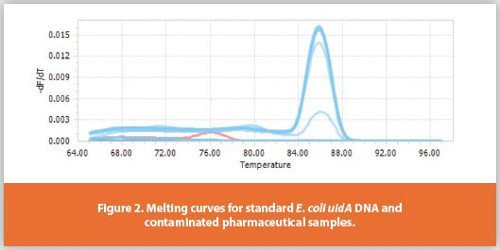
Previous studies on PCR analysis detected E. coli in pharmaceutical products using gel electrophoresis.9-12 Furthermore, quantification of the target gene was not possible. However, in this study SYBR green I, a common double-stranded binding dye, was used to detect and quantify the amplified uidA fragments in DNA samples within the same reaction mix. Gel electrophoresis analysis for RT-PCR was not needed. However, during this study it was performed for verification purposes. The wide variety of pharmaceutical delivery systems, e.g., tablets, liquids, creams, tested in this study were contaminated with different bacterial contaminants to demonstrate the ability of the RTPCR assay to detect E. coli, a common USP indicator, in the presence of other bacterial species.
Faster detection and identification of E. coli contamination is extremely important since some E. coli strains not only spoil the products compromising efficacy and safety but also produce a wide variety of factors that enhance the pathogenicity of the different strains.6 Dispensing products contaminated with E. coli to patients can result in several life-threatening infections such as sepsis, gastrointestinal disorders, septicemia, and pneumonia. Rapid assessment of quality control allowing the expeditious implementation of corrective actions enhances process control during manufacturing of non-sterile pharmaceutical products.
References
- Friedman, R.L. 2005. Aseptic processing contamination case studies and the pharmaceutical quality system. PDA J. Pharma. Sci. and Technol. 59:118-126.
- Jimenez, L. 2007. Microbial diversity in pharmaceutical product recalls and environments. PDA Journal of Pharmaceutical Science and Technology 61:383-399.
- Sutton, S.W., Jimenez L. 2012. A review of reported recalls involving microbiological control 2004-2011 with emphasis on FDA considerations of “objectionable organisms”. American Pharmaceutical Review 15:42-57.
- Essam Eissa, M. 2016. Distribution of bacterial contamination in non-sterile pharmaceutical materials and assessment of its risk to the health of the final consumers quantitavely. Beni-Suef University Journal of Basic and Applied Sciences 5:217-230.
- Bej, A.K., Dicesare, J.L., Haff, L., Atlas, R.M. 1991. Detection of Escherichia coli and Shigella spp. in water by using the polymerase chain reaction and gene probes for uid. Appl Environ Microbiol. 57:1013–1017.
- Peleg, A.Y., Hooper, D.C. 2011. Hospital-acquired infections due to gram-negative bacteria. N. Engl. J. Med. 362:1804-1813.
- Haque, M., Sartelli, M., McKimm, J., Abu Bakar, M. 2018. Health-care associated infectionsan overview. Infect. Drug Resis. 11:2321-2333.
- Jimenez L. 2011. Molecular applications to pharmaceutical processes and clean room environments. PDA Journal of Pharmaceutical Science and Technology 65:242-253.
- Jimenez, L., Smalls, S., Grech, P., Bosko, Y., Ignar, R., English, D. 1999. Molecular detection of bacterial indicators in cosmetic/pharmaceuticals and raw materials. Journal of Industrial Microbiology and Biotechnology 21:93-95.
- Jimenez, L., Smalls, S., Ignar, R. 2000. Use of PCR analysis for rapid detection of low levels of bacterial and mold contamination in pharmaceutical samples. Journal of Microbiological Methods 41:259-265.
- Karanam, V.R., Reddy, H.P., Subba Raju, B.V., Rao, J.C., Kavikishore, P.B., Vijayalakshmi, M. 2008. Detection of indicator pathogens from pharmaceutical finished products and raw materials using multiplex PCR and comparison with conventional microbiological methods. J. Ind. Microbiol. Biotechnol. 35:1007-1018.
- Ragheb, S.M., Yassin, A.S., Amin, M.A. 2012. The application of uniplex, duplex, and multiplex PCR for the absence of specified microorganism testing of pharmaceutical excipients and drug products. PDA Journal of Pharmaceutical Science and Technology 66:307-317.
- Jimenez, L., Jashari, T., Vasquez, J., Zapata, S., Bochis, J., Kulko, M., Ellman, V., Gardner, M., Choe, T. 2018. Real-Time PCR detection of Burkholderia cepacia in pharmaceutical products contaminated with low levels of bacterial contamination. PDA Journal of Pharmaceutical Science and Technology 72:73-80.
- Wang, Q., Garrity, G.M., Tiedje, J.M., Cole, J.R. 2007. Naïve bayesian classifier for rapid assignment of rRNA sequences into the new bacterial taxonomy. Appl. Environ. Microbiol. 73:5261-7.
- Altschul, S.F., Madden, T.L., Schaffer, A.A., Zhang J., Zhang, Z., Miller, W., Lipman, D.J. 1997. Gaped BLAST and PSI-BLAST: a new generation of protein database search programs. Nucleic Acid Research. 25:3389-3402.
- Ko, S., An, H.S, Bang, J.S., Park, S.W. 2015. An outbreak of Burkholderia cepacia complex pseudobacteremia associated with intrinsically contaminated commercial 0.5% chlorhexidine solution. American Journal of Infection Control 43: 266-268.
- Jimenez, L., Pinto, A., Perez, S., Peca, S., Pincus, L. 2018. Real-Time PCR detection of Staphylococcus aureus in pharmaceutical products contaminated with mixed bacterial cultures. Euro. Pharma. Review 6:28-30.
- Vetrovsky, T., Baldrian. P. 2013. The variability of the 16S rRNA gene in bacterial genomes and its consequences for bacterial community analyses. PLoS ONE. 8(2): e57923. doi:10.1371/journal.pone.0057923.
- Bergthorsson, U., Ochman, H. 1995. Heterogeneity of genome sizes among natural isolates of Escherichia coli. J. Bact. 177:5784-5789.
- Maheux, A.F., Picard, F.J., Boissinot, M., Bissonnette, L., Paradis, S., Bergeron, M.G. 2009. Analytical comparison of nine PCR primers designed to detect the presence of Escherichia coli/Shigella in water samples. Water Res. 43:3019-3028.
- Taskin, B., Gozen, A.G., Duran, M. 2011. Selective quantification of viable Escherichia coli bacteria in biosolids by quantitative PCR with propidium monoazide modification. Appl. Environ. Microbiol. 77:4329-4335.
- Jimenez, L., Scalici, C., Smalls, S., Bosko, Y., Ignar, R. 2001. PCR detection of Salmonella typhimurium in pharmaceutical raw materials and products contaminated with a mixed bacterial culture using the BAXTM system. PDA Journal of Pharmaceutical Science and Technology 55:286-289.