Biologic Drug Product Development (BioDPD), Global Lyophilization Center of Excellence (CoE)
Biologic Drug Product Development (BioDPD)
Drug Product Manufacturing Science and Technology (MSAT)
Introduction
Lyophilization (Freeze Drying) is a drug product manufacturing unit operation, used to stabilize active pharmaceutical ingredients such as proteins, many of which are unstable in the liquid state. It is the most commonly used method of stabilization of pharmaceutical drug products and has been the traditional approach for many years (Tang and Pikal 2004). Lyophilization is a process by which a liquid formulation, usually filled into a container such as a type 1 glass vial, undergoes a series of controlled temperature and vacuum steps, by cooling/heating a shelf with heat transfer fluid or controlling vacuum pressure by using a vacuum pump and nitrogen.
The lyophilization cycle consists of three main stages – Freezing, Primary and Secondary Drying. The liquid formulation is first frozen, usually below the glass transition temperature (Tg’) of the freeze concentrate, after which a vacuum is applied. The shelf temperature is increased to sublime the ice (primary drying), and once all the ice has sublimed, the shelf temperature is further increased to drive off unfrozen water (bound water) by desorption (secondary drying). At the end of secondary drying, the final lyophilized drug product remains, which is usually a uniform, pharmaceutically elegant ‘cake’ with a desired stability profile.
Although there are many attempts to study and propose alternative stabilization techniques such as spray drying (Ali and Lamprecht 2017) or spray freeze drying (Sebastiao, Bhatnagar et al. 2019), lyophilization still remains the leading method, and this is unlikely to change in the near to midterm. In fact the field of lyophilization of pharmaceutical drug products seems to be growing with (a) the increasing complexity of modalities to treat complex diseases and (b) the variants of conventional freeze drying such as active freeze drying in bulk (Touzet, Pfefferle et al. 2018) and continuous freeze drying (Pisano, Arsiccio et al. 2019).
To characterize the stability profile of the drug product, there is a focus on certain attributes, known as critical quality attributes (CQAs). These can include (for protein lyophilized drug product): particle formation, aggregation, activity, cake appearance, residual moisture content, and reconstitution time.
Residual Moisture is a critical quality attribute (CQA) of lyophilized drug product and is important for many reasons:
- Residual Moisture levels are directly related to the stability of the active pharmaceutical ingredient (Hsu, Ward et al. 1992).
- Can be a representation of how well the cycle has been designed, scaled up and performed.
- Residual Moisture may trend as a function of time and storage conditions when the lyophilzed drug product is placed on stability, by virtue of the contribution of moisture from the stopper and also from any phase changes during the storage (e.g. mannitol crystallization or release of water molecules from mannitol hemihydrate formulation during the lyophilization process) (Dixon, Tchessalov et al. 2009).
- Residual Moisture is related to the overall glass transition(Tg) of the dried product, and the Tg directly impacts stability if storage occurs near, at or above Tg (Wang, Tchessalov et al. 2009).
- Residual moisture can also support in predictive stability work.
When considering overall impact of physical state, the residual moisture content and the Tg are inversely proportional. An increasing residual moisture content results in a lower overall Tg, this is due to the Tg’ of unfrozen water of -137°C (Rasmussen and MacKenzie 1971), thereby depressing the Tg of the lyophilized solid. This is important especially when considering stability and storage, because if the Tg depresses close to, or below the storage temperature, there may be an increase in molecular mobility and possible degradation of the protein (Pikal, Dellerman et al. 1992). Therefore, measurement of residual moisture as an output of the lyophilization step, during stability, and understanding its relationship to the physical state of the product in question is crucial.
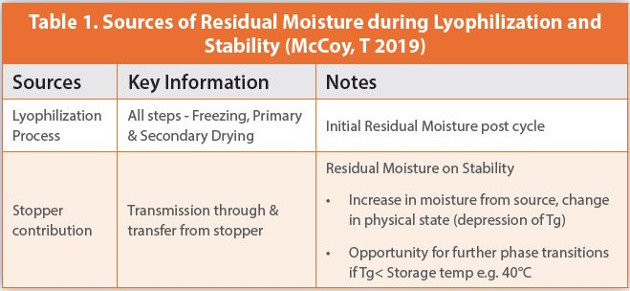
The source of residual moisture during lyophilization is also important to consider. As stated, the lyophilization cycle itself, along with the formulation and any phase changes that occur during the cycle all contribute to the final residual moisture content. In addition, the contribution from the stopper on stability especially at elevated temperature is a factor. The transfer of stopper moisture to the product is critical for products with a low fill volume as the transfer of water from the stopper can lead to considerable increase in residual moisture content. Table 1 represents the source of moisture during the lyophilization step, either as a result of the process itself or contribution from the stopper over time.
Having a good understanding of the residual moisture variability across the freeze dryer for both inter and intra batch, and the impact on both the physical state and overall stability is extremely important. Therefore, a way of measuring residual moisture, which is accurate, representative and high throughput has significant value.
Measurement of Residual Moisture Content
There are a number of ways to measure residual moisture. The most common are as follows:
- Karl Fischer Titration – both for solvent extraction and oven methods
- Thermogravimetric Analysis (TGA) (Matejtschuk et al. 2016)
- Near Infra-red Spectroscopy (NIR) (Clavaud, Lema-Martinez et al. 2019)
- Frequency Modulated Spectroscopy (FMS) (Cook and Ward 2011)
The most common method used to measure residual moisture is by Karl Fischer (KF) Titration. Normally, the method requires some sample manipulation, and even under a controlled inert environment, this may have some impact on the final results as the lyophilized cake can be hygroscopic.
Subscribe to our e-Newsletters
Stay up to date with the latest news, articles, and events. Plus, get special offers
from American Pharmaceutical Review – all delivered right to your inbox! Sign up now!
Thermogravimetric Analysis (TGA) may be used although it is becoming less and less the method of choice. The main challenge with TGA is sample handling and manipulation along with the fact that it is more similar to a loss on drying technique. The quantification of residual moisture is based on weight loss and also can consist of volatiles that are removed at conditions applied to the product. TGA is useful in the R&D setting, but KF would be a better choice for more relative accuracy in measurement.
The challenge with KF is that it is a destructive and low throughput technique where sample preparation and manipulation may be required. Manipulation of the sample should always take place in an inert environment, such as a glovebox, as exposing to the environment of the laboratory while preparing on the bench could impact residual moisture content. The KF method has variations, in that the sample may be reconstituted using organic solvent and injected to the reaction vessel, or the lyophilized cake may be sampled and added directly. When using the KF oven, the scientist can sample the cake, transfer it to a test vial, stopper and crimp and load onto the autosampler for analysis. Lastly, the entire drug product (DP) vial can be analyzed with no manipulation, just heated by the oven and analyzed by piercing a needle through the stopper. The KF method can be restrictive in development and scale up. It is very difficult to robustly sample and measure across batches for residual moisture:
- The traditional methods (TGA, KF) are invasive and sample numbers for testing are limited due to the destructive nature of the assays. These small numbers of vials are not representative of a full commercial manufacturing batch.
- The traditional methods are time consuming and manual sample handling and preparation is required, which can be a source of error.
- The throughput of traditional methods such as Karl Fischer can be slow and uses carcinogenic solvent which poses a safety risk if mishandled.
- It is difficult to generate a data set representative of a batch due to the low throughput and invasive nature of methods, as sample numbers would have to be large and the vials would no longer be available for release.
Non-Destructive Residual Moisture Analysis
The industry is moving quickly towards measurement of residual moisture by non-destructive means. The benefits of moving to nondestructive testing include:
- Efficiency – sample analysis is done in seconds; the sample population for testing can be significantly larger and 100% inspection is feasible.
- Non-destructive – the product may be used for other testing and possibly patient supply – for NIR, product impact is not likely a concern due to short light exposure time.
- Possibility of in-line or at scale.
- Application to map entire development lyophilizer.
- Applicable to commercial manufacturing with no vial loss (no impact to yield).
- Value to batch release in the event of a deviation in the cycle.
The release of lyophilized drug product using a non-destructive method may not be common as yet, but the value of building a data set as part of the characterization or monitoring of an at-scale batch and additionally continuing to collect extremely valuable commercial data, without compromising a single vial, is of real value both to the quality of manufacturing and the bottom line. In a GMP batch release situation where there is a manufacturing deviation holding up the release of a drug product batch, possessing a larger data set to give the engineer or scientist confidence that there is no significant impact on the drug product or projected stability profile is crucial.
Near Infrared Spectroscopy (NIR) is a method that can be used for nondestructive residual moisture analysis of the lyophilized drug product (Clavaud, Lema-Martinez et al. 2019). The basic principle involves shining a beam through the bottom of the vial up into the lyophilized cake. A model can be developed using Karl Fischer as a basis. Once the model is developed, lyophilized vials may be scanned by NIR and the moisture model applied to determine the residual moisture based on peak amplitude. NIR has potential application as a process analytical technology (PAT) (De Beer, Vercruysse et al. 2009) as this method has the potential to be set up in-line on a commercial scale, thereby making 100% in-line residual moisture content analysis across a batch very much a possibility. There is also significant value in using NIR and Raman to complement each other, where NIR is used to measure residual moisture and Raman is used to detect phase composition (De Beer, Vercruysse et al. 2009). Table 2 represents some key details of measurement, detection and calibration of NIR as a method to measure residual moisture of the lyophilized cake.
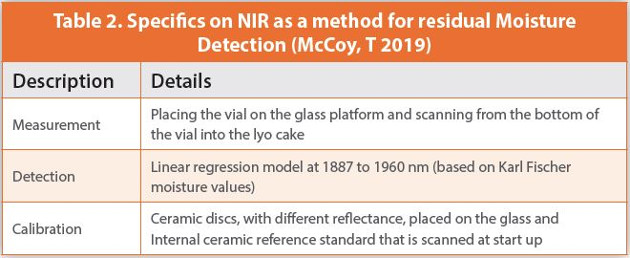
Frequency Modulate Spectroscopy (FMS) (Lin, Hsu et al. 2004) (Cook and Ward 2011) is based on laser absorption spectroscopy. In the case of FMS, the laser is passed through the vial headspace, where the laser is matched to the particular internal vibration frequency of a compound of interest i.e. water, O2, CO2. The pressure in the headspace can be directly measured using FMS, and this can be used to calculate the residual moisture using an algorithm developed from correlating the headspace pressure with residual moisture values as measured by Karl Fischer.
Lastly, Table 4 captures a comparison between the NIR and FMS methods versus Karl Fischer.
The benefits of NIR and FMS over Karl Fischer include speed of testing of a given sample vial, the potential to apply to a much larger sample size and in-line 100% testing at scale – these are all attractive to the industry. Using non-destructive residual moisture techniques in development, scale up and even commercial manufacturing will provide a much better understanding of inter and intra batch performance and possibly enable the reduction or removal of tech batches over time. In addition, NIR and FMS can be combined with other QbD approaches such as modelling of the vial heat transfer coefficient (Kv) and dry layer resistance (Rp). The downside at this point is the fact that calibrating and building the algorithm to measure residual moisture by the non-destructive methods is dependent on traditional means such as Karl Fischer.
There is much to consider when using headspace analysis, such as the partial pressure in the headspace, the impact of cake appearance and whether change in residual moisture can be detected accurately in this case.
Conclusion
Residual moisture is a critical quality attribute that must be monitored post lyophilization cycle and also on stability. It also must be analyzed in conjunction with other product characteristics, such as aggregation and Tg. The traditional methods that are used to measure residual moisture are generally destructive and low throughput. As a result, there is a significant interest in non-destructive testing of residual moisture as part of development, scale up and manufacturing. The possibilities that these methods could offer are significant and it is important to continue the push to understand the benefits and pitfalls of these methods. It is important to understand what improvements can be made to ensure these non-destructive methods are representative of all scenarios. There is an increasing expectation from regulatory agencies to develop and design more quality into the manufacturing process, and with the traditional low throughput moisture methods, this will be a huge challenge.
Acknowledgements
Sanofi colleagues Jean-Rene Authelin, Atul Saluja and Mark Yang for reviewing and providing valuable suggestions.
Global Biological Drug Product Development & Manufacturing (BioDPD&M) and Global Drug Product Manufacturing Science and Technology (MSAT) Organizations
Yatin Gokarn, Global Head BioDPD&M
References
- Ali, M. E. and A. Lamprecht (2017). “Spray freeze drying as an alternative technique for lyophilization of polymeric and lipid-based nanoparticles.” Int J Pharm 516(1-2): 170-177.
- Clavaud, M., et al. (2019). “Near-infrared spectroscopy to determine residual moisture in freeze-dried products: model generation by statistical design of experiments.” J Pharm Sci.
- Cook, I. A. and K. R. Ward (2011). “Applications of Headspace Moisture Analysis for Investigating the Water Dynamics within a Sealed Vial Containing Freeze-dried Material.” PDA J Pharm Sci Technol 65(1): 2-11.
- Cook, I. A. and K. R. Ward (2011). “Headspace Moisture Mapping and the Information That Can Be Gained about Freeze-Dried Materials and Processes.” PDA J Pharm Sci Technol 65(5): 457-467.
- De Beer, T. R., et al. (2009). “In-line and real-time process monitoring of a freeze drying process using Raman and NIR spectroscopy as complementary process analytical technology (PAT) tools.” J Pharm Sci 98(9): 3430-3446.
- Dixon, D., et al. (2009). “The impact of protein concentration on mannitol and sodium chloride crystallinity and polymorphism upon lyophilization.” J Pharm Sci 98(9): 3419-3429.
- Hsu, C. C., et al. (1992). “Determining the optimum residual moisture in lyophilized protein pharmaceuticals.” Dev Biol Stand 74: 255-270; discussion 271.
- Lin, T. P., et al. (2004). “Application of frequency-modulated spectroscopy in vacuum seal integrity testing of lyophilized biological products.” PDA J Pharm Sci Technol 58(2): 106-115.
- McCoy, T. “Measurement of Residual Moisture Content by Non-Invasive Methods.” International Society of Lyophilzation-Freeze Drying (ISLFD) Mid-West Chapter. Chicago, April 2019.
- Pikal, M. J., et al. (1992). “Formulation and stability of freeze-dried proteins: effects of moisture and oxygen on the stability of freeze-dried formulations of human growth hormone.” Dev Biol Stand 74: 21-37; discussion 37-28.
- Pisano, R., et al. (2019). “Achieving continuous manufacturing in lyophilization: Technologies and approaches.” Eur J Pharm Biopharm 142: 265-279.
- Rasmussen, D. H. and A. P. MacKenzie (1971). “The glass transition in amorphous water. Application of the measurements to problems arising in cryobiology.” J Phys Chem 75(7): 967-973.
- Sebastiao, I. B., et al. (2019). “Bulk Dynamic Spray Freeze-Drying Part 2: Model-Based Parametric Study for Spray-Freezing Process Characterization.” J Pharm Sci 108(6): 2075-2085.
- Tang, X. and M. J. Pikal (2004). “Design of freeze-drying processes for pharmaceuticals: practical advice.” Pharm Res 21(2): 191-200.
- Touzet, A., et al. (2018). “Active freeze drying for production of nanocrystal-based powder: A pilot study.” Int J Pharm 536(1): 222-230.
- Wang, B., et al. (2009). “Impact of sucrose level on storage stability of proteins in freeze dried solids: II. Correlation of aggregation rate with protein structure and molecular mobility.” J Pharm Sci 98(9): 3145-3166.
Author Biography
Tim McCoy, M.S. is an Associate Director of Formulation and Process Development, in the Biological Drug Product Development & Manufacturing (BioDPD&M) department at Sanofi R&D Framingham MA. Tim leads a team of scientists in the development of the formulation and manufacturing process for biological drug products from early stage to launch and LCM. Tim also heads the Sanofi BioDPD Global Lyophilization Center of Excellence where the global team work on lyophilization strategy from Phase 1 through to commercial development and launch. During his career, spanning a number of companies and functions, Tim worked on many lyophilized drug product modalities including proteins, vaccines, API, suspensions, both solvent and aqueous based. Tim also has extensive experience in simulation and lyophilization technology.