Small Molecule Design and Development
Abstract
While most of the published work on the impact of residence time distribution (RTD) to pharmaceutical continuous manufacturing (CM) processes focuses on drug product, drug substance process dynamics is vastly different. This work focused on investigating what could represent the major contributor to residence time distribution of a drug substance fully continuous process as it matures: surge vessels. Changes in size or type of surge vessels significantly affect the resulting RTD and this work presents several numerical examples of such an impact. Process development repercussions are also discussed.
Introduction
Continuous manufacturing (CM) is one of the most rapidly growing initiatives aiming to modernize the pharmaceutical industry and streamline regulatory pathways. The ability to produce medicines faster, safely, greenly, modularly, and more flexibly is very appealing to pharmaceutical practitioners.1-3 Production on demand, better control, and lower risk of shortages are factors that also appeals to regulatory bodies.4-7 As CM matures within pharma, it is expected to have a profound impact to both synthetic molecules and biologics.
Over the last few years, drug product CM of small molecules has created tremendous interest and several approved products (e.g., Orkambi® and Symdeko® - Vertex; Prezista® - Janssen; Verzenio® - Eli Lilly).8 Particularly, the concept of residence time distribution (RTD) to design and justify pharmaceutical drug product process control strategy,9,10 understand drug product process performance,11-13 track active ingredient and excipients,14 and create advanced process control approaches,15 has been reported. RTD has been also used for characterization and design of single unit operations16-18 lot genealogy estimation,17 and to investigate perturbation propagation across sections of drug substance continuous processes.18
As shown in Table 1, the dynamics of drug substance and drug product continuous processes are vastly different. This work focuses on identifying main contributors to RTD of fully continuous drug substance processes and the repercussion that those have on process development and commercialization.
Major Contributors to Drug Substance Continuous Process RTD
Due to the number of reactions, workup steps, purification and isolation needs, the average residence time of a drug substance continuous processes may vary from hours to days. As an example, the numerically estimated RTD of a multiple-step drug substance process is presented in Figure 1. That process train is described by the following unit operations/average residence time: Plug Flow reactor (PFR1)/2 h → Online high performance liquid chromatography (HPLC) → Divert valve → Solvent exchange distillation/3 h → Dual surge vessels/23 h → continuous stirred tank reactor (CSTR1)/3 h → Online HPLC → Divert valve → CSTR2/1 h → PFR2/1.5 h → CSTR3/1 h → Online HPLC → Divert valve → Dual surge vessels/3 h → CSTR4/1 h → PFR3/0.5 h → 3 mixer-settlers/1.5 h. Metals used in the reaction steps are removed by liquid-liquid extractions in a series of mixer-settlers. At two intermediate points of the train, dual surge vessels operating in an alternating fashion are installed.
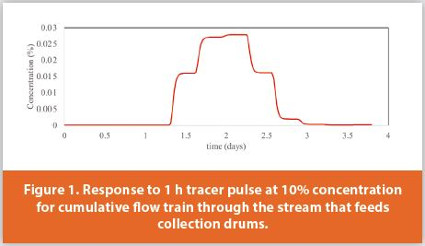
Either a single CSTR configuration or intermediate surge units containing two to three surge tanks (surge farm) that intermittently operate in a fill/empty or fill/empty/idle mode, respectively, are very common in drug substance continuous processes because they allow for partial decoupling of the process (Figure 2). Intermediate surge tanks offer many advantages in a GMP manufacturing environment:
- Decoupling the unit operations, so that one section of the flow train can be down for troubleshooting while the other sections keep running.
- Simplifying startup and shutdown transitions.
- Simplifying automation and control.
- Dampening out process disturbances and facilitating conservative calculations of acceptable magnitude and duration of disturbances.
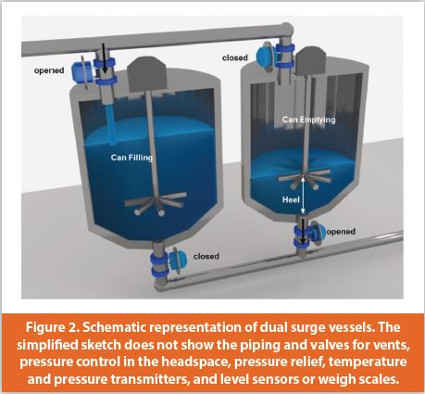
In the investigated example, the two sets of dual surge cans decouple three different synthetic route steps. The first set of dual surge vessels operated at high fill levels, with 23 hours fill and empty times. The desire was to minimize shutdowns of the downstream CSTR1 because of an unstable hold point. Therefore, if the upstream PFR1 or solvent exchange distillation needed to stop for troubleshooting, the downstream CSTR1 could operate for up to 23 hours without needing to be shut down. Furthermore, because the goal was to minimize the chance of shutdowns of CSTR1, the downstream set of dual surge vessels operated with only three hour fill and empty time, although they had higher capacity, in case that downstream unit operations CSTR4, PFR3 or mixer settlers experienced problems. The upstream parts of the process including CSTR1 could continue to run for a time without shutdown gradually filling the second set of dual surge vessels to higher levels. In other words, the liquid levels in the dual surge cans were chosen to favor the operation of CSTR1 without shutdowns.
For the RTD estimation presented here, a virtual twin of the process was developed by connecting model elements representing each unit operation and creating a flowsheet model to represent the actual process (gPROMs Formulated Products). This work reports numerical estimations of RTDs under different scenarios/conditions.
For the investigated process, the concentration of a tracer was estimated at the end of the flow train after a 1 h-duration pulse was imposed at the process feed, starting at t=0. Figure 1 shows that the average residence time under normal operating conditions was approximately two days, it spread between 1.4 and 3.3 days, and the pulse magnitude (initially 10%) was reduced almost three orders of magnitude.
The shape/location of process RTD has important implications for process design, development, and commercialization:
- The ability of the train to dampen disturbances is extremely powerful and it can significantly reduce the probability of diversion events if the magnitude of the perturbation doesn’t exceed undesirable levels.
- If the magnitude of a disturbances does reach undesirable levels and stream diversion is needed to avoid impact the final active ingredient, broader RTDs will require to divert material for longer period than narrower RTDs. For that reason, diversion usually takes place in stations located at earlier points of the train. This strategy differs from drug product processes, as narrower RTD favors diversion at the end of the process.
- In contrast with drug product CM that favor high frequency methods, specificity and sensitivity of process analytical technology (PAT) will be of major importance for drug substance processes (e.g., on-line HPLC).
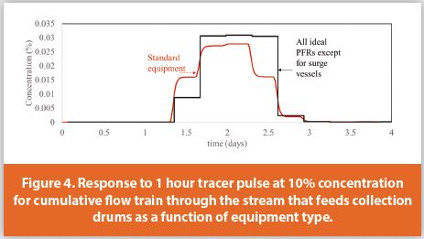
As briefly mentioned before, surge vessels, also known as intermediate storage tanks, buffer tanks, surge drums, accumulators, or inventory tanks are used to partially or fully decouple upstream and downstream units, maintain a smooth continuous operation, and to dampen disturbances.20-23 An inventory of material accumulated at intermediate points of a drug substance CM process prevents brief localized equipment failure from resulting in the immediate shutdown of the entire process. Surge vessels are also used to simplify startup and shutdown transitions, simplify automation and control and cleanouts, and provide valuable time to understand if material can be forward processed. Typically, batch collection vessels and drums help subdivide the plant into smaller but independent sub-processes, while continuously or semi-continuously operated vessels are used for partial decoupling. Economic considerations, broad RTDs and long average residence times tend to support minimizing/eliminating surge vessels. However, this approach is often more difficult from an operational perspective due to poor dynamic controllability of processes. As surge vessels help to simplify the process when it is in development stages or it is being run in the manufacturing plant for the first time, they contribute to a significant portion of the average residence time and spread of the process RTD. As a process becomes more mature, it may be desirable to remove surge vessels to reduce process footprint, decrease the amount of material at risk, tighten RTD, and reduce the number of pumps.
Subscribe to our e-Newsletters
Stay up to date with the latest news, articles, and events. Plus, get special offers
from American Pharmaceutical Review – all delivered right to your inbox! Sign up now!
In the example investigated in Figure 1, ~70% of the average residence time of the process is contributed by intermediate surge vessels. Figure 3 shows that as the amount of material accumulated in the first set of intermediate surge vessels is reduced from 69 Kg to 43.2 Kg (reducing the fill and empty time from 23 h to 14 h), both the average residence time and RTD spread reduce significantly.
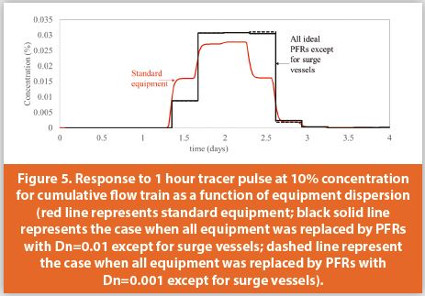
Surge vessels affect the overall train dispersion. Figure 4 compares the impact on the resulting RTD when standard equipment is used (i.e., combination of PFRs and CSTRs) with the case where all pieces of equipment were replaced by PFRs, except for the surge cans. While the shape of the distribution changed, there was not significant changes to either process average residence time or the overall spread of the RTD, highlighting the critical contribution of surge vessels to the overall RTD of drug substance processes. Figure 5 and Figure 6 show that increasing the axial dispersion of those replaced PFRs one order of magnitude (i.e., from a dispersion number Dn of 0.001 to 0.01) did not impact the estimated RTD noticeably. Dn (defined as Dax/uL where Dax is the axial dispersion, u is the fluid velocity, and L is the equipment length) is an important parameter for reactor design. D/uL 0.01 versus 0.001 may have impact on conversion versus distance in the reactor. However, Figure 5 and Figure 6 show that the effect of dispersion of individual pieces of equipment is negligible on the overall RTD, as the overall spread is mostly governed by the dynamics of surge vessels.
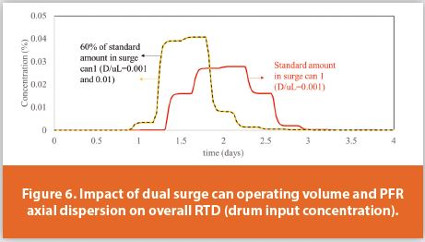
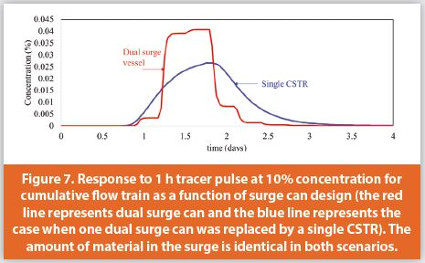
The surge vessel design/configuration also affects the overall RTD. Figure 7 compares the RTD of a train that uses dual surge cans vs a single CSTR with the same total amount of accumulated material. As expected, a broader distribution was obtained when using a single surge vessel with CSTR residence time distribution.
Conclusions
The effect of surge vessels on RTD may be more or less pronounced than that in the example investigated here depending on the process surge capacity (i.e., amount of material accumulated in surge vessels). Due to the multiple practical benefits that surge vessels offer though, large contributions to the overall RTD by surge vessel is expected to be a relatively common situation for drug substance processes. Surge vessel design needs to be guided by typical engineering considerations such as maximum storage capacity and minimum tank stirred volume, but also RTD-impacted aspects such as desired dampening effect and chemical stability of streams. Surge vessel size and location contributes to the control strategy helping design diversion points and define overall PAT needs among others. Numerical models to determine RTD is a great tool to help guide those design decisions.
Acknowledgements
The authors would like to acknowledge Dr. Christopher Polster for his foundational work on gPROMS model library and Dr. Bret Huff and Dr. Paul Collins for their support with CM.
References
- Wilburn K. Business Case for Continuous Manufacturing of Pharmaceuticals 2010. Master Thesis, Massachusetts Institute of Technology.
- 2. De Belder L. Analyzing the Business Case Drivers for Continuous Drug Product Manufacturing 2016 Pharmaceutical Online. Available at: https://www.pharmaceuticalonline.com/doc/analyzing-the-business-case-drivers-for-continuous-drug-product-manufacturing-0001. Accessed Jan 9, 2020.
- Shanley A. Continuous Manufacturing: Separating Hype from Reality. Pharmaceutical Technology 2017, 2017(2):8-1,31.
- Chatterjee S. FDA Perspective on Continuous Manufacturing. IFPAC Annual Meeting 2012. Available at: https://www.fda.gov/media/85366/download. Accessed Jan 9, 2020.
- Lee S. Modernizing the Way Drugs Are Made: A Transition to Continuous Manufacturing. News & Events for Human Drugs 2017. Available at: https://www.fda.gov/drugs/news-events-human-drugs/modernizing-way-drugs-are-made-transition-continuousmanufacturing. Accessed Jan 9, 2020.
- Kopcha, M. Advanced Manufacturing for Small Molecule and Biotechnology Drug Products: Improved Quality for the Sake of Patients. PD2M Future of Pharmaceutical Manufacturing Workshop 2019, Washington D.C. USA
- Osorio, M. Th New launch: CBER’s Advanced Manufacturing for Complex Biologics Products. Commercializing Continuous Processes in Pharma, 4th Annual Summit 2020, Boston, USA.
- (1) Vertex, Continuous OSD Manufacturing – PharmEng (ISPE), Available at: https://ispe.org/pharmaceutical-engineering/ispeak/continuous-osd-manufacturing-productpatient-perspective; (2) Vertex receives FDA approval for continuously (Symdeko – Vertex’s 2nd CM approval)… - PharmTech, Available at: http://www.pharmtech.com/vertexreceives-fda-approval-continuously-manufactured-drug-product; (3) Janssen, EMA approves Janssen’s Prezista – European Pharmaceutical Review, available at: https://www.europeanpharmaceuticalreview.com/news/62587/ema-continuous-manufacturing/. FDA approves Janssen’s Prezista – PharmTech.com, http://www.pharmtech.com/fdaapproves-tablet-production-janssen-continuous-manufacturing-line; (4) FOYA 2017, Lilly is the 2017 Overall Winner, Pharmaceutical Engineering 2018;38(1):24-30.
- Almaya A, De Belder L, Meyer R, Nagapudi K, Lin H, Leavesley I, Jayanth J, Bajwa G, DiNunzio J, Tantuccio A, Blackwood D, Abebe A. Control Strategies for Drug Product Continuous Direct Compression – State of Control, Product Collection Strategies and Startup/Shutdown Operations for the Production of Clinical Trial Materials and Commercial Products. J Pharm. Sci. 2017;106:931-943.
- Manley l, Shi Z. Characterizing drug product continuous manufacturing residence time distributions of major/minor excipient step changes using near infrared spectroscopy and process parameters. Int. J. Pharmaceutics. 2018;551:60-66.
- Gao Y, Muzzio F, Ierapetritou M. A Review of the Residence Time Distribution (RTD) Applications in Solid Unit Operations. Powder Techn 2012;228:416-423.
- Wesholowski J, Berghaus A, Thommes M. Investigations Concerning the Residence Time Distribution of Twin-Screw-Extrusion Processes as Indicator for Inherent Mixing. Pharmaceutics 2018;10(4):207-217
- Wesholowski J, Berghaus A, Thommes M. Inline Determination of Residence Time Distribution in Hot Melt Extrusion. Pharmaceutics 2018;10(2):49-59
- Engisch W, Muzzio F. Using Residence Time Distributions (RTDs) to Address the Traceability of Raw Materials in Continuous Pharmaceutical Manufacturing. J Pharm Innov 2016;11:64-81
- Bhaskar A, Singh R. Residence Time Distribution (RTD)-Based Control System for Continuous Pharmaceutical Manufacturing Process. J. Pharm. Innov. 2019;14:316-331.
- Johnson M, May S, Calvin J, Remacle J, Stout J, Diseroad W, Zaborenko N, Haeberle B, Sun W, Miller M, Brennan J. Development and Scale-Up of a Continuous, High Pressure, Asymmetric Hydrogenation Reaction, Workup, and Isolation. Org. Proc. Res. Dev. 2012;16:1017-1038.
- Reizman B, Cole K, Hess M, Burt J, Maloney T, Johnson M, Laurila M, Cope R, Luciani C, Buser J, Campbell B, Forst M, Mitchell D, Braden T, Lippelt C, Boukerche M, Starkey D, Miller R, Chen J, Sun B, Kwok M, Zhang X, Tadayon S, Huang P. Small-Volume Continuous Manufacturing of Merestinib. Part 2. Technology Transfer and cGMP Manufacturing. Org Proc. Res. Dev. 2019;23(5):870-881
- Cole K, Groh J, Johnson M, Burcham C, Campbell B, Diseroad W, Heller M, Howell J, Kallman N, Koenig T, May S, Miller R, Mitchell D, Myers D, Myers S, Phillips J, Polster C, White T, Cashman J, Hurley D, Moylan R, Sheehan P, Spencer R, Desmond K, Desmond P, Gowran O. Kilogram-Scale Prexasertib Monolactate Monohydrate Synthesis Under Continuous-Flow cGMP Conditions. Science 2017;356(6343):1144-1150.
- May S, Johnson M, Buser J, Campbell A, Frank S, Haeberle B, Hoff man P, Lambertus G, McFarland A, Moher E, White T, Hurley D, Corrigan A, Gowran O, Kerrigan N, Kissane M, Lynch R, Sheehan P, Spencer R., Pullet S, Stout J. Development and Manufacturing GMP Scale-Up of a Continuous Ir-Catalyzed Homogeneous Reductive Amination Reaction. Org Proc. Res. Dev. 2016;20:1870-1898
- Faanes A, Skogestad S. A systematic approach to the design of buffer tanks. Comp. Chem. Eng. 2000, 24, 1395-1401.
- Karimi I, Reklaitis G. Optimal Selection of Intermediate Storage Tank Capacity in a Periodic Batch/Semicontinuous Process. AIChE J 1983, 29(4), 588-596.
- Hiester A, Melsheimer S, Vogel E. Sizing and Locating Surge Capacity. Chem. Eng. Comm. 1990, 89, 87-100.
- Aufuldish E, Melsheimer S. Optimization of Surge Capacity. Chem. Eng. Comm. 1994, 129, 251-275.