Introduction
Throughout the last few years, there has been a growing trend in counterfeit cases involving pharmaceutical products.1 The United States Food and Drug Administration (FDA) defines a counterfeit drug product as a fake medicine that is contaminated, contains the wrong active ingredient, contains no active ingredient, or contains incorrect dosages of an active ingredient.1 Counterfeit drugs are illegal and directly threaten patient safety, either through lack of efficacy or exposure to hazardous materials. According to the Pharmaceutical Security Institute (PSI), there were 4,405 pharmaceutical crime incidents worldwide in 2018, an increase of 102% over the past five years and a trend that is expected to increase.2
Organizations such as the FDA and PSI continually monitor reports of suspect counterfeit cases in order to protect patients from the threat of counterfeit drugs. Statistics are typically used to highlight trends in product types or geographic locations where counterfeiting is most common.2 Pharmaceutical manufacturers are also finding ways to fight back by adding holograms, barcodes, encryptions, and other overt and covert features into their products for authentication purposes.3 In addition, pharmaceutical manufacturers also employ analytical techniques such as spectroscopy and chromatography to determine the chemical composition of suspected counterfeits.
There are a variety of analytical techniques used for the detection and confirmation of counterfeit drugs. Gas chromatography (GC), high performance liquid chromatography (HPLC), and other techniques such as capillary electrophoresis and x-ray diffraction have all been used to detect the presence or absence of a correct active pharmaceutical ingredient (API). However these techniques are slow, hazardous solvents and extensive sample preparation are often involved, and a high level of training is required to operate the analytical instrumentation.4 Faster, less labor intensive techniques for the chemical screening of suspected counterfeit products include molecular spectroscopy, such as Fourier Transform Infrared (FTIR), Near Infrared (NIR), and Raman spectroscopy.4 These lab-based instruments can detect the presence or absence of the API and confirm the authenticity of drug products. However since these are laboratory based instruments, they still require proper facilities and analyst training to operate.
Despite the many efforts developed to detect counterfeit drug products and report them to authorities in a timely manner, a need still exists for a fast, accurate, and portable counterfeit screening device to help ensure supply chain integrity and patient safety. This article discusses a portable Raman spectroscopic technique which can be deployed in the field to detect counterfeit drugs. Suspect tablets can be directly scanned by the Raman laser and spectral fingerprints are then used to confirm whether a tablet is authentic or counterfeit. During this study, data generated by a portable Raman instrument is compared to data obtained from a benchtop FT-Raman instrument.
Raman Spectroscopy
Raman spectroscopy is a nondestructive analytical technique used for the molecular fingerprinting of pharmaceutical drug products. It is based on the principle of inelastic light scattering, where a quanta of energy is transferred from the incident excitation laser to the target molecule.5 This quanta of energy gained by the target molecule is vibrational energy and is referred to as the Raman frequency. This inelastic Raman scattering carries information on the molecule’s bond vibrations and functional groups, which may ultimately be used for spectral fingerprinting.5 One advantage of the Raman technique is that, in many cases, no sample preparation or dilution is needed because of low scattering efficiency. Most pharmaceutical oral dosage forms may be run in their native state. In addition, the source laser can be directly focused through many types of drug product packaging, which makes analysis simple, fast, and safe.6
A Raman spectrometer consists of several main components including an excitation source, fi ber optics, filters, mirrors, an interferometer or dispersive element such as a holographic grating, and a detector depending on which type of instrument is used. A laser serves as the excitation source and is used to induce Raman scattering. Shorter excitation wavelength lasers result in higher Raman signal because the Raman scattering is inversely proportional to the 4th power of laser wavelength,7 but they suff er from undesired fluorescence. The laser wavelengths of the benchtop and portable Raman instruments used in this article were 1064 nm and 785 nm, respectively. The laser scattered energy is then transmitted, collected, and filtered. Filtering eliminates unwanted Rayleigh scattering, when photons are scattered at the same energy as the incident photons, or anti-Stokes, when photons have more energy than the incident photons.7 The remaining light is passed through a dispersion system such as an interferometer or holographic grating and a detector captures the scattered light. Counterfeit pharmaceuticals comprised of a variety of tablet coatings and varying amounts of active and inactive ingredients may all be detected and differentiated using Raman spectroscopy.8 The acquired spectra can include peaks arising from functional groups such as NH, CH, and OH found in pharmaceutically active ingredients and inactive excipients, both of which contribute to the spectral fingerprint for that product.9 This technique has also been used to combat counterfeiting of pharmaceutical biologic products, such as injectable liquids, by using FT-Raman instruments9 and the drop coat deposition confocal Raman (DCDCR) spectroscopic technique.10
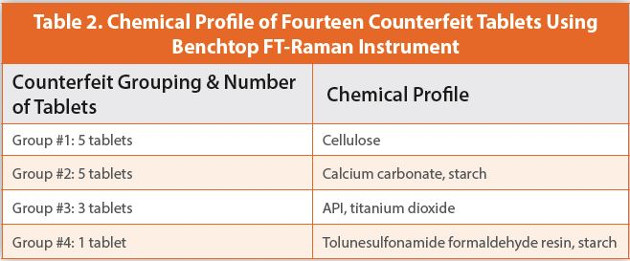
Benchtop Versus Portable Raman Spectroscopy
There are many pros and cons of both the benchtop and portable Raman spectrometers. The main advantages of the benchtop instrument is a high-powered laser, variety of sampling accessories to customize applications, and the high spectral resolution of the acquired spectra. Benchtop instruments tend to have better signalto-noise and less interferences from fluorescence due to higher excitation wavelength. There are also large spectral library databases that can assist in identifying compounds quickly and accurately. The disadvantages of benchtop instruments include the inability to test samples in the field and the resulting long cycle times when sending samples to an equipped laboratory.11 Benchtop instruments require samples to be sent to a centralized testing lab which is an issue for countries which have legal restrictions preventing the export of counterfeit samples. The high powered lasers in these instruments could be a safety concern as well. Portable instruments could minimize these limitations because of their small size, ability to generate fast results, and lower laser power.
Portable instruments can help expedite counterfeit identification and quickly screen drugs for product authenticity in places such as warehouses, airports or law enforcement offices. Similar to benchtop instruments, portable devices also have large library databases available for identifying and screening counterfeits. The disadvantages of portable Raman devices include lower-excitation wavelengths, which can result in fluorescence interferences.11 Nonetheless, despite the two instruments having their own strengths and weaknesses, both Raman techniques are effective for counterfeit drug screening. The goal of this article was to compare the performance of a benchtop and a portable Raman instrument for the detection of counterfeit pharmaceuticals. The table below outlines the specifications for both of the Raman instruments used in this article.
Subscribe to our e-Newsletters
Stay up to date with the latest news, articles, and events. Plus, get special offers
from American Pharmaceutical Review – all delivered right to your inbox! Sign up now!
As outlined in Table 1, the benchtop instrument has a higher laser power and wider spectral range but is limited by bulky dimensions. In contrast, the portable instrument is more versatile and compact in size but is limited by a weaker laser power and a shorter spectral range.
Experimental
For both the benchtop and portable Raman instruments, the same spectral analysis software program was used to analyze fourteen counterfeit tablets, representing four different counterfeit groups, against a variety of known authentic tablets. The counterfeits were first scanned on the benchtop instrument and the chemical compositions were determined via library database correlations (see Table 2). The same counterfeits were scanned a second time on the portable instrument and chemical compositions were determined again. The chemical profile determined for the counterfeit tablets by both instruments were then compared.
Table 2 outlines the four diverse counterfeit groups screened on the benchtop instrument. Each group contains counterfeit tablets that had the same chemical profile. The groups differ based on the different excipients in each product. Group 3 is the only set of counterfeit tablets to contain an API, but the formulation did not match any authentic tablets. Group 3 is also the only group to contain titanium dioxide, an inactive excipient used in tablet coatings. The chemical identity of the counterfeits detected by the portable instrument was compared to these groups.
Unlike the benchtop instrument, the portable device was not previously used to screen these types of counterfeit products and therefore a method was created to screen the tablets. Different batches of authentic and counterfeit tablets were used in the method during qualification so the variables were independent. Authentic tablets were analyzed on the portable instrument and collected as spectral references which were stored as a new method in the library database. Qualification of this method was confirmed when authentic products were detected and a passing result was displayed and counterfeit products were rejected when a failing result was displayed. After qualification, this method was used to screen the fourteen counterfeit tablets. When the counterfeits did not match the spectra of any authentic products in the method, the counterfeit tablets underwent a library database search in the portable spectrometer software to determine their chemical identity. The chemical identification determined by the portable instrument was then compared against the chemical identification of the same counterfeits by the benchtop instrument.
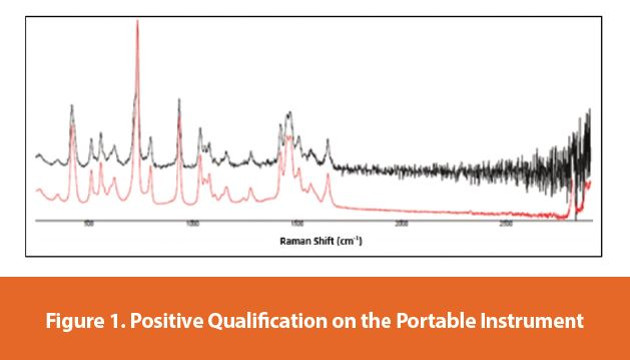
Method Qualification Results for Portable Instrument
A method was created on the portable instrument to screen the counterfeit tablets. The goal of the qualification was to confirm that authentic samples would be detected and displayed as real products while counterfeit samples would be rejected and displayed as fake products. It was also expected to have the ability to identify the chemical components screened against the portable instrument once rejected by the method.
Figure 1 shows an authentic product saved in the method (red) compared against a scan of a real pharmaceutical tablet (black). The portable device was able to determine that the analyzed tablet was authentic and displayed a passing result with a p-value above 0.05. A p-value equal to or above 0.05 generates a “Pass” result and indicates the sample spectrum is similar to the authentic spectrum. Passing results were generated for all authentic products tested.
Figure 2 shows a spectral reference for an authentic product saved in the method (red) compared against a scan of a known counterfeit tablet (black). The portable instrument was able to determine that the tablet screened was not authentic and displayed a failing result with a p-value below 0.05. A p-value below 0.05 generates a “Fail” result and indicates the sample spectrum is significantly different from the authentic spectrum. Failing results were shown for all counterfeits tested. Overall, the method was able to differentiate between authentic and counterfeit pharmaceuticals.
Raman Spectroscopy Results
Raman spectral fingerprinting was measured on the benchtop FTRaman and portable Raman instruments for the fourteen counterfeit tablets. The spectra comparisons are shown below.
Raman spectra were collected between 150-1500 cm-1 on the benchtop FT-Raman instrument. The red spectrum represents an authentic product while the various other colored spectra represent counterfeit tablets from each of the four groups. None of the spectra of the counterfeit tablets have identical fingerprints when compared against the authentic tablet. With the exception of group 3, all counterfeit tablets failed to exhibit the API peak at approximately 720 cm-1, which is used as a marker since it is selective for the API. Despite detecting API, group 3 was confirmed as a counterfeit since titanium dioxide was detected and is not present in the authentic formulation. None of the counterfeit tablets displayed major correlations to the authentic tablet. The FT-Raman benchtop instrument was able to distinguish the difference between authentic and counterfeit products by detecting differences in their Raman signals.
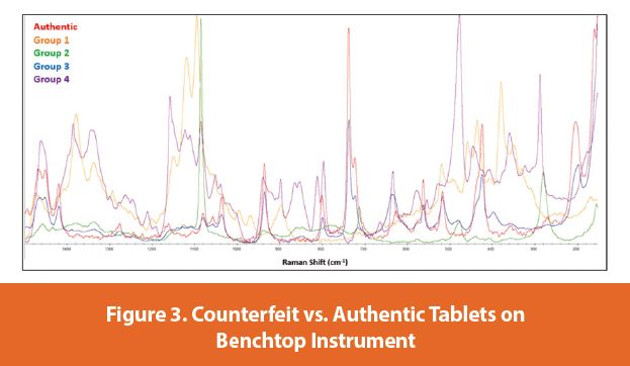
Raman spectral fingerprinting was measured between 250-1500 cm-1 on the portable Raman instrument for the fourteen counterfeit tablets. The red spectrum represents an authentic product while the various other colored spectra represent counterfeit tablets from each of the four groups. Similar trends were observed such as failure to exhibit the API marker peak at approximately 720 cm-1 and the detection of titanium dioxide that is not present in the authentic formulation. However, fluorescence was observed in all counterfeit screenings because of the lower excitation wavelength (785 nm) compared to the benchtop FT-Raman instrument excitation laser wavelength (1064 nm). Overall, the portable instrument was able to distinguish the difference between authentic and counterfeit tablets, similar to the benchtop instrument, by detecting differences in Raman spectra.
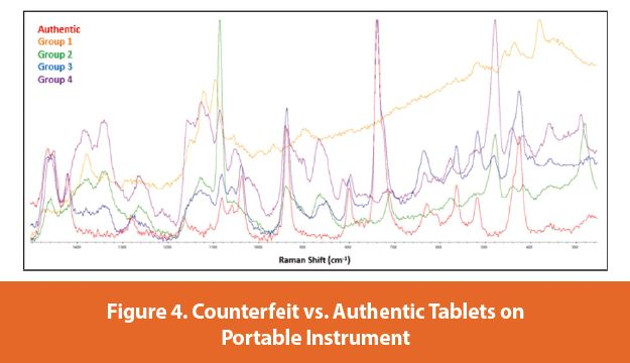
Table 3 compares the chemical composition of the counterfeit tablets as detected by both the benchtop and portable Raman instruments. The chemical composition determined by the portable instrument was comparable to that of the benchtop instrument. Both instruments were able to detect active ingredients, excipients, and coating material. The only difference was that the benchtop instrument was very easily able to detect the API through the titanium dioxide tablet coating material. The portable instrument could not detect the API as easily as the benchtop instrument which resulted in the tablet being cut in half to obtain Raman signal from the core. Taking a scan from the tablet core as opposed to the tablet coating will result in less background signal from the coating material. Overall, the composition of the counterfeit tablets were measured comparably using both instruments, confirming that the portable Raman spectrometer is a competitive and effective tool for the detection of counterfeit drugs in the field.
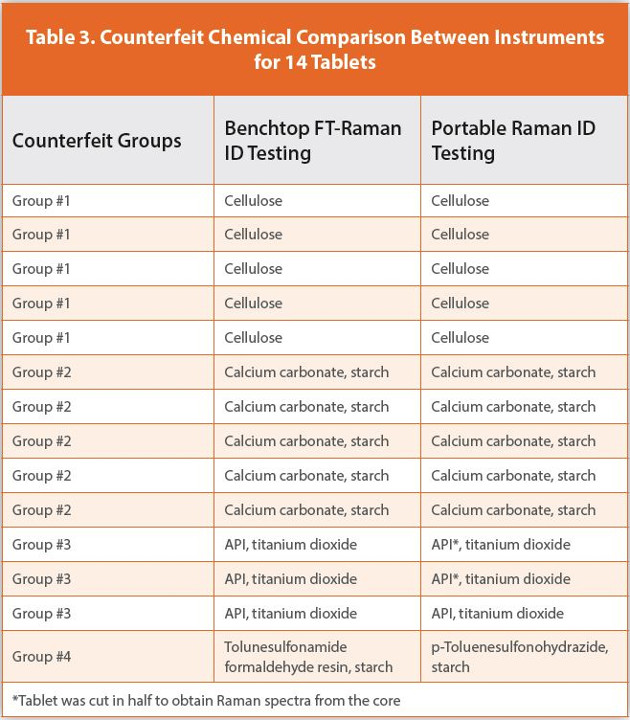
Conclusion
The portable Raman spectrometer was able to positively detect counterfeit tablets and determine their chemical composition comparably to the benchtop FT-Raman spectrometer. All 14 counterfeit tablets had similar chemical profiles when classified by the two instruments. Both instruments were able to detect active ingredients, excipients, and coating material. However, the portable instrument may require taking spectra from the core if high background signal is encountered from coating components. The technology behind Raman spectroscopy has rapidly evolved over the years and there are many benefits of these portable units.12 It has been shown that portable instruments can perform similarity to the larger, more expensive benchtop instruments and could easily be deployed into the field in hopes of making counterfeit detection and identification easier and faster.
References
- United States Food & Drug Administration (FDA). Counterfeit medicine information page. Available at: https://www.fda.gov/drugs/buying-using-medicine-safely/counterfeitmedicine. Accessed January 15, 2020.
- Pharmaceutical Security Institute. Counterfeit incident trends information page. Available at: https://www.psi-inc.org/incident-trends. Accessed January 15, 2020.
- Hemalatha, R., Srinivasa Rao, A., Electronic solutions for combating counterfeit drugs. J. Pharm Bioallied Sci. 2015;7(3):230-232
- Kovacs, Stephanie, Hawes, Stephen, Maley, Stephen, Mosites, Emily, Wong, Ling, Stergachis, Andy. Technologies for detecting falsified and substandard drugs in low and middle-income countries. PLoS One. 2014;9(3):e90601.
- Peters, Jeremy, Luczak, Anna Ph.D., Ganesh, Varsha, Park, Eugene, Kalyanaraman, Ravi Ph.D. Raman spectral fi ngerprinting for biologics counterfeit drug detection. American Pharmaceutical Review. 2016;19(2):46-51.
- Kalyanaraman, Ravi, Chauhan, Mitesh, Madarang, Clemonne John, Ribick, Michael. Screening and detecting counterfeit biologics drugs. BioPharma Asia. 2013; 58-64
- Mettler Toledo. Raman spectroscopy information page. Available at: https://www.mt.com/gb/en/home/applications/L1_AutoChem_Applications/Raman-Spectroscopy.html. Accessed January 15, 2020.
- Neuberger, Sabine, Neusub, Christian. Determination of counterfeit medicines by Raman spectroscopy: systematic study based on a large set of model tablets. J Pharma and Biomedical Analysis. 2015;112:70-78.
- Kalyanaraman, Ravi, Dobler, George, Ribick, Michael. Portable Spectrometers for Pharmaceutical Counterfeit Detection. American Pharmaceutical Review. 2010;13(3):38-45.
- Peters, Jeremy, Luczak, Anna Ph.D., Ganesh, Varsha, Park, Eugene, Kalyanaraman, Ravi Ph.D. Protein secondary structure determination using drop coat deposition confocal Raman (DCDCR). Spectroscopy. 2016;31(10):31-39.
- Degardin, Klara, Guillemain, Aurelie, Roggo, Yves. Comprehensive study of a handheld Raman spectrometer for the analysis of counterfeit of solid-dosage form medicines. Journal of Spectroscopy. 2017;4:1-13.
- Luczak, Anna PhD, Kalyanaraman, Ravi PhD. Portable and Benchtop Raman Technologies for Product Authentication and Counterfeit Detection. American Pharmaceutical Review. 2014;17(6):76-80
Author Biographies
Brittany Handzo, is an analytical chemist at Bristol-Myers Squibb Company in the Global Quality Analytical Science and Technology group within Global Product Development and Supply. Her roles within GQAS&T include development and validation of particle size method along with working in the lab to test and screen of suspect and counterfeit pharmaceuticals. She received her Master’s Degree in Chemistry from Seton Hall University under the direction of Dr. Nicholas Snow where she studied gas chromatography. She also has a Bachelor’s Degree in Chemistry from Fairleigh Dickinson University where she conducted research in high performance liquid chromatography.
Anna Luczak, PhD, is a Senior Scientist I at Bristol-Myers Squibb Company in the Global Analytical Technology group with Global Manufacturing and Supply and is currently working on development of novel spectroscopic method for detection of counterfeit biologics. She received her Ph.D. in Analytical Chemistry from New Jersey Institute of Technology under the direction of Prof. Zafar Iqbal where she explored Raman spectroscopy for various pharmaceutical applications. Before joining BMS in 2014, she worked at Johnson and Johnson where she was responsible for process improvements of existing products including scale-up and transfer to the supplier site.
Scott Huffman, is an associate director at Bristol-Myers Squibb Company in the Global Quality Analytical Science and Technology group within Global Product Development and Supply. He is currently the operations manager for the forensic laboratory located at the New Brunswick, New Jersey site. Scott received his Bachelor of Science degree from Rutgers University in 1992 and has been with Bristol-Myers Squibb since 2001. For seventeen years Scott worked within the Analytical Development organization of Bristol-Myers Squibb where he was responsible for the development and validation of analytical methods for the identification of pharmaceutical materials and products. His current work has been focused on developing and implementing new and novel techniques to detect pharmaceutical counterfeits & to support particulate and foreign matter characterization in samples from manufacturing processes. His interests are mainly in using vibrational spectroscopic techniques such as Raman, Mid-Infrared (IR) and Near-Infrared (NIR) for pharmaceutical counterfeit detection and product authentication. His group also extensively employs microscopy (optical, Raman, IR and SEM) and elemental analysis (EDS) in support of this work. He leads a team of scientists which provide analytical support to product quality, corporate security and manufacturing operations groups.
Jeremy Peters, is a Scientist II in Global Analytical Technology group in Global Manufacturing and Supply at Bristol-Myers Squibb Company. He is involved in developing new and novel techniques for Biological Drug Product Characterization and Structure determination. He is also responsible for particulate and foreign matter characterization in manufacturing investigation using IR, Raman and SEM/EDS techniques. Prior to joining Global Analytical Technology, Jeremy worked at the Syracuse site of BMS where he was responsible for QC testing of Raw Materials used for Drug Substance batches. Jeremy has a Bachelor’s degree in Chemical Engineering from the New Jersey Institute of Technology.
Ravi Kalyanaraman, PhD, is a Director at Bristol- Myers Squibb Company in the Global Analytical Technology group with Global Manufacturing and Supply. He received his Ph.D. from the University of Idaho in 1995 and did his postdoctoral work at the University of Puerto Rico. He served as a faculty member in the department of chemistry at Bemidji State University in Minnesota from 1996 to 2001. He has been with Bristol-Myers Squibb since 2002. His laboratory work in the past with Bristol-Myers Squibb was primarily in developing and validating chromatographic methods for Quality control laboratories. In the last ten years he has focused on developing new and novel techniques to detect pharmaceutical counterfeits and raw material identification using vibrational spectroscopic techniques, such as Raman, mid-, and near-infrared (NIR). Currently, he leads a team of analytical scientists that are involved in the forensic and manufacturing investigation for products received from product complaints, corporate security and also from various manufacturing sites including third party manufacturing. Also, his team has developed new and novel Raman spectral fingerprint techniques for biologics drugs which can be used to screen counterfeit biologics. He also leads a team of subject matter experts who are involved in QC method remediation, re-validation and periodic method assessment.