Abstract
Ensuring the quality of pharmaceutical products is paramount for bringing them to market. This calls for analytical methods with high accuracy, specificity, and reproducibility. Nonlinear optical spectroscopy is an emerging toolbox in this context. This article provides an overview of the working principles and features of four techniques that have proven to be suitable for pharmaceutical analysis: second harmonic generation (SHG), coherent anti-Stokes Raman scattering (CARS), two-photon excitation laser-induced fluorescence (2P-LIF), and stimulated Raman scattering (SRS).
Introduction
With the invention of the laser in the 1960s, spectroscopy utilizing nonlinear optical effects became feasible. Over the last decades, these methods have been developed to become true alternatives to the established analytical tools like spontaneous Raman scattering and Fourier-transform infrared spectroscopy. In many cases, they even outperform their linear counterparts as they provide features such as advanced specificity and background suppression. In the pharmaceutical sector, nonlinear optical methods are gaining an ever-increasing interest, in particular for the analysis of solid materials that can be the product, raw material, or intermediates.
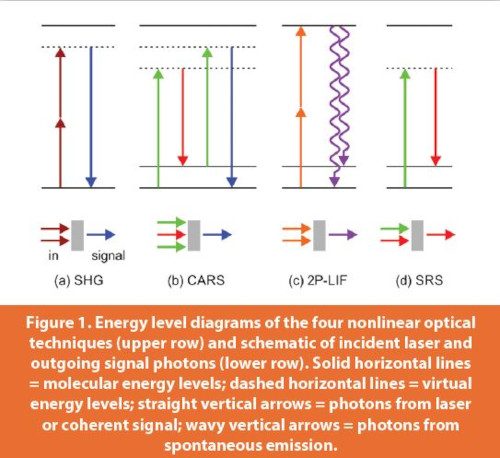
Nonlinear optical effects arise from the interaction of multiple incident photons, typically coming from laser sources, with a material. The use of multiple photons makes the application of nonlinear techniques more complicated as several laser beams have to be overlaid precisely in space and time in order to produce the desired signal. On the other hand, we gain molecular specificity and reduce unwanted background signals, which can be a challenge in the conventional analysis. For instance, elastically scattered laser light and laser-induced fluorescence can make it impossible to acquire meaningful Raman spectra, but an equivalent background-free spectrum may be recorded with a nonlinear Raman technique.
Nonlinear Optical Techniques
There are four nonlinear optical methods that are frequently applied in the analysis of pharmaceuticals: second harmonic generation, coherent anti-Stokes Raman scattering, two-photon laser-induced fluorescence, and stimulated Raman scattering. Figure 1 illustrates the underlying light-matter interactions in their corresponding energy level diagrams. For a more complete picture and theoretical underpinnings, the reader is referred to the literature, e.g. Refs. 1,2, as only a brief outline is given in the following:
Second Harmonic Generation (SHG) is a second-order optical process, see Figure 1a. Two photons from a laser interact with the material at the same time to yield a signal at the twofold frequency of the incident laser light. The process does not require a molecular resonance. This is good and bad: the experiment does not need many adjustments to the sample under investigation, but on the other hand, the signal provides very limited information about the chemistry as it is not molecule-specific. However, a strong benefit of the method is that the signal is exclusively generated at macroscopic or microscopic interfaces.
Therefore, it allows us to identify crystals and phase boundaries in a pharmaceutical composite matrix like a tablet.
Coherent Anti-Stokes Raman Scattering (CARS) is a nonlinear counterpart of conventional Raman spectroscopy. It is a third-order process in which a first pair of photons coherently drives vibrational modes of the molecules of interest and a third photon probes this coherence, see Figure 1b. Due to the molecular resonance of the first two photons, the method is highly specific. When ultrashort laser pulses (femtosecond duration) are used, the probe beam can even be delayed in time to scan the vibrational dynamics of the excited modes. A disadvantage of the method is its experimental complexity, but there is also substantial benefit. First and foremost, there is excellent chemical contrast and high sensitivity. Moreover, the coherent (laser-like) nature of the signal allows an effective suppression of interferences.
Stimulated Raman Scattering (SRS) is another nonlinear variant of conventional Raman spectroscopy. Like in Raman spectroscopy, a pump laser irradiates the sample, but instead of relying on the spontaneously emitted Raman signal, there is a second laser beam to induce emission, see Figure 1d. This second beam, which is often referred to as a Stokes beam, is resonant with the Raman signal. As we have discussed for CARS, SRS is molecule-specific and provides high sensitivity. In fact, there are two ways to operate an SRS experiment: either the gain at the Stokes wavelength or the loss at the pump wavelength is monitored giving rise to Stimulated Raman Gain (SRG) and Stimulated Raman Loss (SRL) modes, respectively.
Subscribe to our e-Newsletters
Stay up to date with the latest news, articles, and events. Plus, get special offers
from American Pharmaceutical Review – all delivered right to your inbox! Sign up now!
Two-Photon Excitation Laser-Induced Fluorescence (2P-LIF) has a special place on the list of nonlinear optical methods. It combines a nonlinear two-photon absorption process with a spontaneous signal emission, see Figure 1c. The previously described methods share the coherent nature of the emitted signal. This is different in 2P-LIF, where the signal is emitted isotropically. The benefit of the two-photon absorption is that no or negligible background signals are produced as excitation is made with low-energy photons (typically in the near-infrared). The advantage of the isotropic signal is that the method can be easily applied using conventional spectroscopic microscopes. The sensitivity of the two-photon excitation variant, however, is lower than the conventional laser-induced fluorescence technique.
Nonlinear Techniques in the Pharmaceutical Sector
The above-described methods have found numerous interesting applications in the pharmaceutical sector. For recent overviews, see Refs. 3,4.
A common use of SHG is to identify the crystals of active pharmaceutical ingredients (API) in an amorphous powder matrix or to monitor the development of API crystals during production or different types of treatment. For example, Sarkar et al.5 recently demonstrated the monitoring of crystal growth rates of individual crystallites as well as the direct detection of nucleation events. They used the antiviral drug ritonavir as a test case.
CARS and SRS provide an enhanced chemical contrast as they probe molecular vibrations. Example applications include the determination of the API distribution in tablets, but even drug release from dissolving carriers can be monitored.6 The nonlinear Raman techniques can also be used to study the drug delivery in tissue, which makes them attractive tools for the development of new drugs, e.g. to tackle new diseases such as COVID-19.
2P-LIF is often combined with other techniques in order to acquire multimodal data. In particular, its combination with SHG is beneficial to add chemical contrast to the predominantly interface-sensitive measurement. For instance, this feature was utilized by Toth et al.7 to study the model API griseofulvin in a complex matrix of excipients.
Conclusion
In conclusion, nonlinear optical spectroscopy is a growing toolbox with numerous rapid, species-specific, and highly sensitive methods for pharmaceutical analysis. These techniques are particularly interesting for a variety of applications such as product analysis and the study of drug release and delivery. They can provide chemical contrast as well as phase contrast with extremely high spatial and temporal resolution.
References
- R.W. Boyd, Nonlinear Optics, 4th edition, Elsevier, 2020.
- C.J. Lee, C.J. Strachan, P.J. Manson, T. Rades, Characterization of the bulk properties of pharmaceutical solids using nonlinear optics – a review, Journal of Pharmacy and Pharmacology 59 (2007) 241-250.
- S. Vanden-Hehir, W.J. Tipping, M. Lee, V.G. Brunton, A. Williams, A.N. Hulme, Raman imaging of nanocarriers for drug delivery, Nanomaterials 9 (2019), 341, 19pp.
- P.D. Schmitt, Recent advances in nonlinear optical analyses of pharmaceutical materials in the solid state, Molecular Pharmaceutics 14 (2017) 555-565.
- S. Sarkar, Z.T. Song, S.R. Griffin, N. Takanti, A.D. Vogt, A. Ruggles, G.D. Danzer, G.J. Simpson, In Situ Crystal Growth Rate Distributions of Active Pharmaceutical Ingredients, Molecular Pharmaceutics 17 (2020) 769-776.
- M. Windbergs, M. Jurna, H.L. Offerhaus, J.L. Herek, P. Kleinebudde, C.J. Strachan, Chemical imaging of oral solid dosage forms and changes upon dissolution using coherent anti-Stokes Raman scattering microscopy, Analytical Chemistry 81 (2009) 2085-2091.
- S.J. Toth, J.T. Madden, L.S. Taylor, P. Marsac, G.J. Simpson, Selective imaging of active pharmaceutical ingredients in powdered blends with common excipients utilizing two photon excited ultraviolet-fluorescence and ultraviolet-second order nonlinear optical imaging of chiral crystals, Analytical Chemistry 84 (2012) 5869-5875.
Author Biography
Prof. Dr. Johannes Kiefer is Chair Professor and Head of the Engineering Thermodynamics department at the University of Bremen, Germany. In addition, he holds a guest professorship of the Erlangen Graduate School in Advanced Optical Technologies (SAOT) at the University Erlangen-Nuremberg, Germany. His research interests are the areas of developing and applying spectroscopic techniques for the characterization of advanced materials and processes.