Introduction
Rapid microbiological methods continue to advance, with novel technologies appearing on the marketplace albeit more orientated towards general research or to the food quality sector rather than conventional pharmaceutical applications. This article, in acknowledging that pharmaceuticals continue to move forwards, examines some recent advances, drawing on technologies that have not been specifically developed for pharmaceuticals, but which have the potential to be applied in the future. This application could either be for research purposes or as a quality control test. The reason for this lateral approach is because rapid methods in the pharmaceutical sector are covered by such luminaries as Miller, Cundell and Moldenhauer; and also because it is interesting to consider which applications of microbiological technology might be influencing the area of pharmaceuticals and healthcare next. Because of this, some of the information presented will be simply informative as to current developments, other aspects may assist with later selection and adoption of the technologies.1 What pharma is good at is applying research and technology in novel ways.
Rapid Microbiological Methods
While the term ‘rapid microbiological methods’ is commonplace, it is perhaps better to use ‘rapid and alternative microbiological methods’ within the pharmaceutical space, especially when alternatives to compendial methods are being discussed. Certainly the only limitation on alternative methods is the pace of technological development itself, as Miller points out: “It is fair to state that virtually any microbiological test that is used to support the development, manufacture, and release of non‐sterile pharmaceutical drug products can be replaced by one or more of the available rapid methods available to the industry.”2 It also stands there is no universal consensus on the definition of ‘fast’. This may mean slightly faster or obtaining the result within a working day shift. The rapid concept also requires further probing when considering a new technology. For example, does the concept include time-consuming steps such as enrichment cultures and isolation of pure cultures, or is ‘rapid’ simply a reference to the instrument run-time once all preparatory work has been completed?
Antimicrobial Resistance
Emerging molecular and phenotypic rapid tests can lead to improved antimicrobial stewardship interventions. In the medical field, this is by reducing both the time to effective treatment and the misuse of antibiotics.3 In the pharmaceutical context, such technologies can assist with the development of new antimicrobials, through antimicrobial susceptibility testing. Approaches include polymerase chain reaction (PCR) based techniques, mass spectrometry, microarrays, microfluidics, cell lysis-based approaches and whole-genome sequencing. Each technology has demonstrated an ability to detect resistance in various bacterial species. Digital PCR in particular allows for better quantification of target molecules present in samples.4 However, there are variations in terms of possessing sufficient sensitivity and specificity to accurately determine resistance.
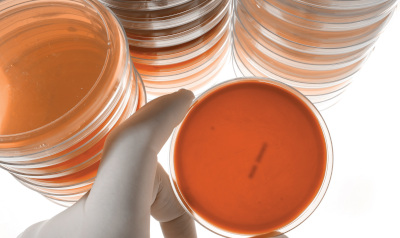
An example of a technology with high specificity and sensitivity is the Accelerate PhenoTest® BC Kit, in which fluorescence in situ hybridization (FISH) is used to provide a microorganism identification within 90 minutes is combined with more established morphokinetic cellular analysis techniques, which to provide a phenotypic antibiogram with in a timeframe of 6–7 hours.5 Other emerging technologies, which do not require a preparation step, are methods based on nucleic acid amplification tests (NAAT), nucleic acid hybridization or immunodiagnostics. It has been established that a short cultivation with a pre-determined antibiotic load followed by NAAT (like isothermal amplification) can reveal antimicrobial resistance, and provide an estimate of the minimal inhibitory concentration (MIC) for screened antimicrobials against a specific organism of interest.6
Complications include assessing what is the primary microbiological epidemiology that will present a risk to the patient. This is necessary for technologies based on DNA analysis, since assessment requires selecting the appropriate panel of probes required to assess specific subsets of pathogens. A secondary area is with defining the approaches required to implement novel rapid tests into pre-existing laboratory workflows and algorithms.
When considering novel methods to assist with antimicrobial research and development, the following questions may be suitable for pitching to the vendor of a new item of technology:7
- Is it generally applicable or suitable only for one specific organism at a time or multiple?
- What is the capacity? How many organisms/agents per hour can be processed?
- Has the technology been validated against reference methods?
- Are there any reference installations?
- Is scientific literature available?
- When will it be on the market?
Lab-on-a-Chip
Lab-on-a-chip systems are technologies that combine multiple laboratory functions into a single integrated circuit (i.e., ‘the chip’). These devices are just a few millimeters to a few square centimeters in shape. The objective is to achieve automation and high-throughput screening.8 The use of lab-on-a-chip technology is useful for pharmaceutical applications, including the use of microorganisms in drug discovery, as well as for some types of toxicological tests that target microbial toxins. A further microbiological application is with the so-called “bacteria-on-a-chip” approach. This combines sensors made from living cells with ultra-low-power electronics that can convert the bacterial response into a wireless signal that can be read by a smartphone. This type of technology has a potential role in wearable devices for the assessment of patient health, especially gastrointestinal problems or for examining the effect of a new drug treatment on the intestinal microbiome.
Subscribe to our e-Newsletters
Stay up to date with the latest news, articles, and events. Plus, get special offers
from American Pharmaceutical Review – all delivered right to your inbox! Sign up now!
While miniaturization creates the conditions for high throughput, it also sets challenges pertaining to the standardization of the necessary conditions that enable similar growth states and culture densities to more established methods. Hence, a concern with lab-on-a-chip methods relates to the small sample size and whether such a sample is truly representative of the whole bacterial population in a sample. This problem is sometimes addressed by analyzing a large number of individual samples (down to the single-cell level).9 A related problem is that growth-based analysis may fail if the growth rate is very low or the microbes cease to grow due to the lack of specific growth factors and it often stands that favorable growth conditions are complicated to establish for miniaturized set-ups.
Biosensors
Biosensors are devices that measure biological or chemical reactions by generating signals proportional to the concentration of an analyte in the reaction. Sensors are potentially capable of assessing bacterial membranes, morphology, metabolism, movements, mass, heat production and nucleic acid content.10 One area of application of relevance to pharmaceuticals is with the real-time monitoring of microbial viability in biofilms, an application that could be harnessed for assessing water systems.
One challenge with microbiology is with the detection of low numbers of microbial cells. A biosensor like Field Effect Enzymatic Detection (has the capability to detect low bacterial concentrations in a liquid sample (below 10 CFU/mL). This technology uses an electrical field, active between a working electrode and a biocatalytic output current, enabling a direct detection of bacteria. The device deploys horse radish peroxidase as a redox source in the sandwich hybridization complex. As this type of technology is commercialized it could play a role in real-time assessments of Water-for-Injection distribution loops or storage tanks.11
Flow Cytometry
In addition to biosensors, more established techniques such as flow cytometry and immuno-magnetic separation can be utilized as rapid, quantitative, and versatile methods to assess microbial numbers in water. This approach can be combined with nucleic acid probes and immune fluorescence in order to provide an estimate of viability (something which has hampered the traditional application of flow cytometry for the assessment of water systems).12 This assessment of viability is similar to adenosine tri-phosphate (ATP) analysis, in terms of providing an estimate of active and viable biomass.13
In terms of more specific screening of organisms of concern in water, detection has been reported based on lateral-flow test strip (LFTS) immunoassays (such as when screening for coliforms). This is a form of immunochromatography, involving the migration of an antigen, or antigen–antibody complexes.14
Difficult to Culture Organisms
Standard approaches for identifying microorganisms in the pharmaceutical setting are based on such methods as microscopy, culture, phenotypic methods, genotypic methods, and nucleic acid amplification. In general, such techniques are reliant upon culture-based profiling. Not only does culturing take time, culture-based methods are not suitable for organisms rendered unculturable due to their metabolic state or which are simply non-culturable against standard culture media. While methods like MALDI-TOF (a proteomics method);15 PCR, and hybridization-microarrays (which can detect the expression of thousands of genes at the same time), have helped to increase turnaround and , arguably, the accuracy of the result these methods work best with known, culturable organisms. In turn, next-generation DNA sequencing as part of metagenomic and bioinformatics strategies have helped to broaden sensitivity to a wider array of organisms. One downside with these second-generation sequencing technologies is their cost, coupled with long sequencing durations. Greater scope may come from third generation sequencing technology. This includes real-time nanopore sequencing.16 Whilst firmly in the clinical camp, such technology may be able to aid pharmaceuticals with difficult to culture or identify organisms.
Summary
This article has outlined some of the technologies emerging as rapid microbiological methods and has considered where these technologies have the potential to be applied to the pharmaceutical sector. In doing so, the article has also touched upon some of the complications and challenges involved in establishing such methods.
This leads into the importance of undertaking microbiological method validation, which is essential for replacing a compendial method with a new method. This is either in relation to the qualification of the method itself or in relation to testing samples against a specific method.17 With this it is important to plan and to devise a suitable validation protocol, outlining acceptance criteria and the way that the validation will be executed.
Validation aside, whether some of the technologies outlined become embraced by pharma remains to be seen. What is clear is that the progression of rapid microbiology methods continues unabated.
References
- Sandle, T. (2014) Approaching the Selection of Rapid Microbiological Methods, Journal of Validation Technology, 20 (2): 1-10)
- Miller, M.J. (2019) Rapid Microbiological Methods. In Roesti, D. and Goverde, M. (Eds.) Pharmaceutical Microbiological Quality Assurance and Control: Practical Guide for Non‐ Sterile Manufacturing, Wiley: https://doi.org/10.1002/9781119356196.ch13
- Giacobbe, D. R. et al (2020) Rapid microbiological tests for bloodstream infections due to multidrug resistant Gram-negative bacteria: therapeutic implications, Clinical Microbiology and Infection, 26 (6): 713-722
- Li Y., et al (2017) Loop-mediated isothermal amplification (LAMP): A novel rapid detection platform for pathogens. Microb. Pathog. 107:54–61. doi: 10.1016/j.micpath.2017.03.016
- Pancholi P, et al. (2018) Multicenter evaluation of the Accelerate PhenoTest BC kit for rapid identification and phenotypic antimicrobial susceptibility testing using morphokinetic cellular analysis. J Clin Microbiol 56: pii: e01329–17
- van Belkum, A., et al. (2019). Developmental roadmap for antimicrobial susceptibility testing systems. Nat. Rev. Microbiol. 17, 51–62. doi: 10.1038/s41579-018-0098-9
- Kahlmeter, G. (2016). Rapid Phenotypic Susceptibility Testing. in Symposium Lecture in Session: New and Rapid Detection of Antimicrobial Resistance. ECCMID Symposium 2016 on Bacterial Susceptibility & Resistance. Amsterdam, The Netherlands. Available online at: https://www.escmid.org/escmid_publications/escmid_elibrary/material/?mid=33585
- Volpatti, L. R. and Yetisen, A. K. (2014). Commercialization of microfluidic devices. Trends in Biotechnology. 32 (7): 347–350
- Bhattacharyya, R., et al (2017). Rapid phenotypic antibiotic susceptibility testing through RNA detection. Open Forum Infect. Dis. 4, S33–S33. doi: 10.1093/ofid/ofx162.082
- Butini, M. E., et al. (2018). Real-time antimicrobial susceptibility assay of planktonic and biofilm bacteria by isothermal microcalorimetry. Adv. Exp. Med. Biol. 1214, 61–77. doi:10.1007/5584_2018_291
- Shi, X., et al (2018). Culture-free bacterial detection and identification from blood with rapid, phenotypic, antibiotic susceptibility testing. Sci. Rep. 8:3416
- Bonadonna, L. (2019) Innovative analytical methods for monitoring microbiological and virological water quality, Microchemical Journal,150: 104160
- Hammes, F., Goldschmidt, F., Vital, M., Wang, Y. & Egli, T. Measurement and interpretation of microbial adenosine tri-phosphate (ATP) in aquatic environments. Water Res. 44, 3915–3923 (2010).
- Tominaga, T. (2019) Rapid detection of coliform bacteria using a lateral flow test strip assay, Journal of Microbiological Methods, 160: 29-35
- Cheng, K., et al (2016) Recent developments of mass spectrometry and proteomics applications in identification and typing of bacteria, Proteomics Clin. Appl. 10,: 346−357
- Gu W, et al (2018) Clinical metagenomic next-generation sequencing for pathogen detection. Annu Rev Pathol-Mech Dis 14(1); 317-336
- Miller, M.J. A (2015) Fresh Look at USP <1223> Validation of Alternative Microbiological Methods and How the Revised Chapter Compares with PDA TR33 and the Proposed Revision to Ph. Eur. 5.1.6. American Pharmaceutical Review. 18(5): 22-35