Prachi Khamkar - Project Mentor, Pharmaceutical Manufacturing Operations, CiREE Edutech, Pune, Maharashtra, India
Debarshi Kar Mahapatra - Department of Pharmaceutical Chemistry, Dadasaheb Balpande College of Pharmacy, Maharashtra, India
Abstract
The pharmaceutical sector is on the brink to join the fourth industrial revolution. It also allows clinicians to develop better-performing medical equipment by providing new methods to personalize treatment. Fused deposition modeling melts extruded drug-loaded filaments into any dosage form, enabling the creation of flexible dosage forms with a variety of active medicinal components and controlled drug release kinetics - in other words, personalized medicine. It is a manufacturing method that builds a three-dimensional item layer by layer from a 3D digital model created using computer-aided design software The goal of this project was to provide an update on this technology while also addressing its difficulties. The pharmaceutical business and compounding pharmacies working together seems to be the best approach to make this technology a reality. Pharmaceutical companies can mass-produce drug-loaded filaments with the required quality and safety assurances, while digital pharmacies can turn the filaments into customized medication based on particular prescriptions. This review will assist you in understanding 3D Printing technology, particularly fused deposition modeling, and its recent advancements in the pharmaceutical industry.
Introduction
Many technological advancements have propelled the healthcare industry to the next level. 3D printing (3DP) is however one invention that is improving the health sector. 3DP has a wide range of applications, including pharmaceuticals, orthopedics, and dentistry. It also enables clinicians to develop better-performing medical equipment for personalized treatment. This innovative method aided the medical sector in areas such as dosage form modification, medical implants, and the dentistry industry during the pandemic. Simultaneously, the pandemic showed how cutting-edge technology like 3DP might help patients with their essential requirements. As a result, there is a shift toward a more forward-thinking approach to healthcare.
3DP is a method that builds a three-dimensional item layer by layer from a 3D digital model created using computer-aided design (CAD) software. 3DP offers many benefits over conventional rapid prototype methods, including lower material costs and the ability to enable a quicker iteration mode.1 The rapid advancement and development of 3DP technology has elevated it from a quick prototype tool to a preferred production method in a variety of industries.2-4 As a result, 3DP is regarded as a highly cost-effective manufacturing method, particularly when creating complicated items. It is currently the preferred approach for producing custom-designed items that would otherwise be prohibitively costly and time-consuming to manufacture using traditional manufacturing methods such as injection molding, machining, and casting.5,6 3DP technology is being utilized in a variety of sectors, including architecture,7 construction, automotive, food, fashion, medical,1 and many more.
Fused Deposition Modelling
Fused deposition modeling (FDM) was invented by Scott Crump in 1988, and Stratasys Corporation Ltd. was founded in 1989. FDM is an additive material extrusion technique that uses thermoplastic polymers to build objects. The unique design of this platform allows for the use of 3D printing in fields such as engineering and medical, broadening the applications. FDM has a simple fundamental aspect yet can produce complicated shapes. It is a melt extrusion method in which a feedstock filament is supplied by a device controlled by an electric motor, as illustrated in the Figure 1.
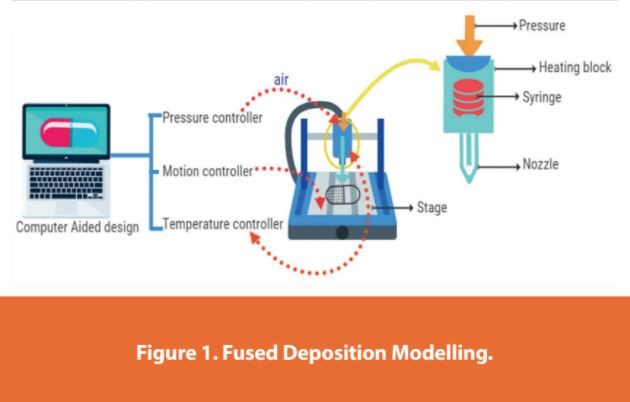
The filament is melted using a heated liquefier. Stepper motors move the liquefier/print head unit over a platform. The melted filament is pushed via the liquefier to the nozzle, which deposits the melt on the platform in the XY plane (fixtureless worktable). Following the completion of deposition at each consecutive cross-section, the platform or print head travels down or up along the Z-axis by precisely one layer thickness. As a result, the 3D structure is built layer by layer. This procedure is repeated until the component is completed. There is a buildup of material along the part’s edge at the start of the FDM process, and subsequently in the internal area of the outline. To pack the component according to the reaction needed, a certain number of outlines is necessary.
There are three important parameters to be considered before printing such as making sure the physical and chemical quality of the filament are covered by material-specific parameters. They analyze the print process feasibility. Printing temperature and infill density, for example, are operation-specific factors that affect the final quality and dissolving behavior of the objects.
Recent Innovations In Fused Deposition Modelling
Hot-melt extrusion coupled with FDM 3DP an advanced pharmaceutical application for personalized drug delivery
FDM is the most widely used 3DP process, in which thermoplastic filaments are used as a starting material and the material is extruded in successive layers above its melting temperature to make a 3D object. These filaments could be made using the Hot-Melt Extrusion (HME) process. The benefit of using HME to make polymer filaments for FDM printing is that a homogeneous solid dispersion of two or more pharmaceutical excipients, i.e., polymers, can be made, and a thermostable drug can even be added to the filament composition, which is otherwise impractical with other techniques (Figure 2).8 By incorporating HME methods into 3DP filament production, medication bioavailability and solubility may be improved, as well as drug release can be sustained for longer periods of time. When it comes to 3DP medical implants, the latter is particularly interesting. In recent years, there has been a growing interest in using a continuous manufacturing technique to design and produce pharmaceutical goods, in order to guarantee high quality and effectiveness while reducing batch-to-batch variability. HME and FDM technologies may be coupled to provide a single continuous processing platform. This research considers how HME and FDM operate, and these two technologies may be integrated for advanced pharmaceutical applications.
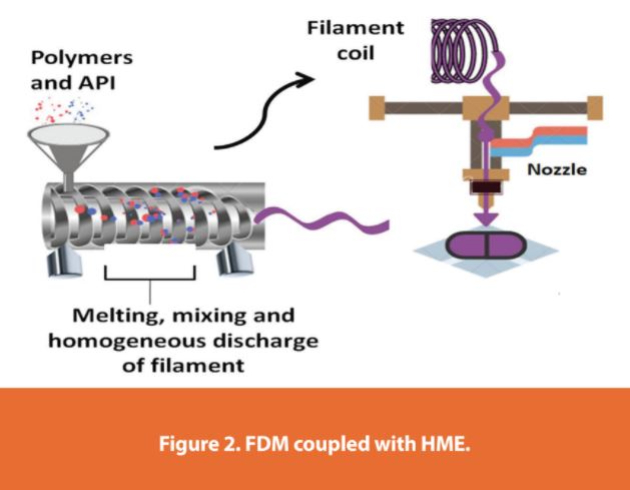
FDM for drug-loaded cardiovascular prosthesis design
Cardiovascular disorders are the primary cause of mortality in the world. Several cardiac implants, such as stents, vascular grafts, and valvular prosthesis, have been created to fight these disease and enhance the quality and span of life. The installation of these vascular prostheses carries risk such as infection and the development of blood clots. Medicated vascular prostheses had previously been utilized to circumvent these constraints. Using FDM technology, Niamh et al. propose a 3DP technique for developing medicated vascular prostheses. Rifampicin (RIF) was considered as a model molecule because it would be utilized to prevent infection in vascular graft prostheses.9 HME was used to mix thermoplastic polyurethane (TPU) with RIF to create filaments with RIF concentrations varying from 0% to 1% w/w. These materials could provide RIF release for 30 to 80 days. Furthermore, RIF-containing TPU-based materials inhibited the development of Staphylococcus aureus. Even TPU-based polymers with RIF concentrations of 0.1% w/w exhibited this behavior. Even after 30 days of RIF release, TPU containing 1% w/w RIF exhibited antibacterial capabilities. These techniques were also utilized to make TPU filaments containing dipyridamole. Finally, vascular grafts containing both medicines were created utilizing a dual extrusion 3D printer (Figure 3).
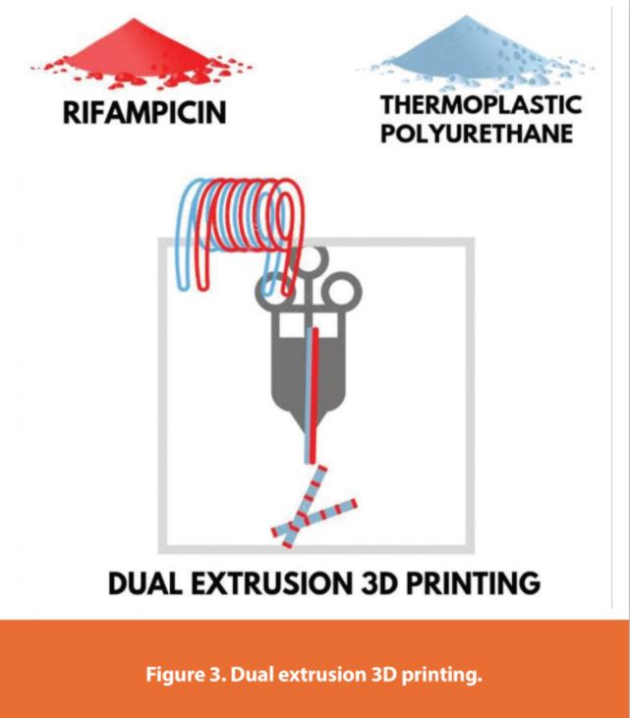
Drug-loaded 3D printed multi-shell rods for retinal vascular disease local delivery
Due to the short half-life of medicines, therapeutic treatment for retinal vascular disorders requires frequent intravitreal injections, placing health and economic difficulties on patients. As a result, an improved drug delivery method that can extend therapeutic action while minimizing side effects was required. Jae et al., in this study, created a core/shell drug-loaded rod (drug rod) that can administer two kinds of medicines; dexamethasone (DEX) and bevacizumab (BEV) from a single implant. By employing a polymeric shell and a hydrogel core, the coaxial printing method enabled BEV and DEX to be delivered with distinct kinetics at the same location. The proposed printing method makes it easier to make drug rods of different sizes and drug concentrations, and the multi-layered structure enables the dual drug-delivery system’s release profile to be adjusted. A small-gauge needle was used to inject the rod into the rat vitreous. As a result, scientists were able to show that the rods were more convenient than conventional intravitreal BEV injections in repressing inflammation and long-term subjugation of retinal neovascularization, implying that they could be used as a treatment modality for a variety of retinal vascular disorders.
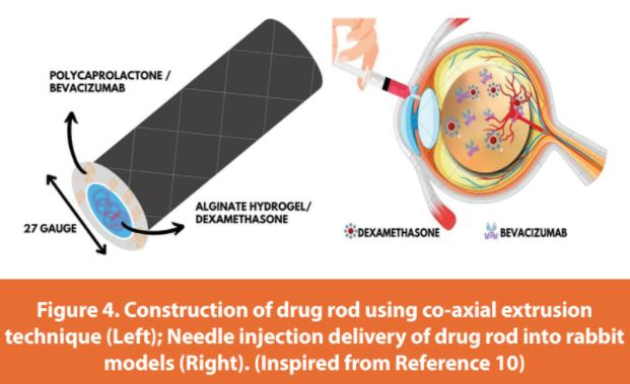
Haptic evaluation of Braille-encoded 3D Printed Intraoral Films
The pharmaceutical industry has recently discovered 3DP, which can be used for personalized dosage and complex formulation designs. Another benefit of 3DP is that it can imprint haptic indicators right on the surface of the compounds, making them touchable. This approach may generate novel design concepts for specific populations, such as visually impaired people, in order to identify the optimal treatment plan.11 In this regard, the FDM technique was investigated for making intraoral films containing Braille letters on the open location. The films have a drug-loaded section and a backing layer, which are similar in architectural characteristics for buccal delivery. The model medicine was ketoprofen, and the polymer matrix for both sections was a hydrophilic polymer termed hydroxypropyl methylcellulose. The Braille-encoded words were created on top of the backing layer, using the pharmaceutical Braille Marburg Medium spacing standard.
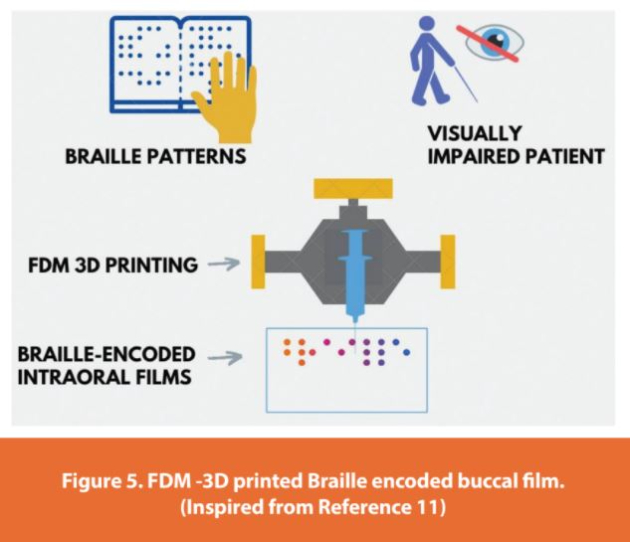
In addition, changes to the usual spacing and dimension parameters were made to test the FDM process’ accuracy and repeatability (Figure 5).
To evaluate the readability of the 3D-printed Braille-encoded text, the produced films were submitted to a haptic assessment research with the help of visually challenged people. The results of the research showcased the FDM’s capability in using innovative manufacturing ideas to craft unique and personalized treatments while offering targeted services that recognize specific groups of people, as can be seen in individuals with vision impairment.
Challenges
Heat processing the formulation during FDM 3DP compromises medication stability. In fact, initial use of this technique revealed stability problems for thermosensitive medicines.12 Plasticizers or polymers with low glass transition temperatures have been used to reduce nozzle temperatures.13-16 For pharmaceutical applications, a more precise and sensitive heater temperature control is required. Excessive heat may cause changes in the viscoelasticity of the polymer, affecting drug control release and, ultimately, drug stability.
Commercial filaments have a fixed 1.75 mm diameter, another issue with certain FDM 3D printers is the lack of nozzle size flexibility. However, because of the viscoelastic properties of polymeric materials, a wide range of polymeric materials are extruded for pharmaceutical applications, resulting in diameter variations. In such circumstances, a printer with flexible nozzles is required.17 The printing time of a batch may be sped up by using an efficient FDM printer with multiple nozzles, or devices with different APIs could be produced without changing filaments throughout the process.18 There are already commercial models that fulfill such requirements, namely the Stacker S4® (Stacker Corp. Ltd., USA), an industrial multi-nozzle 3D printer capable of producing four objects in one go.
Although hot-melt extrusion is a well-established industrial drug manufacturing technique, the finer points of fabricating printable filaments require more research. Thermal and rheological tests should be carried out to assess component compatibility as well as their appropriateness for extrusion processing and 3DP FDM. After that, stability tests should be carried out to establish the product’s shelf life. The manufacture of filaments from polymers with defined solubilization properties can result in a variety of customized drug release patterns (slow, fast, pH-dependent, etc.).
The diameter of the filament is critical for the printing process; an incorrect size might cause the extrudate to clog or result in a decreased feeding rate.19 Some hygroscopic polymers can induce diameter expansion, making filament passing through the printer mechanism more difficult.20 The extruder dye end is prone to distortion due to the heating process, and it is recommended that a pulley with a cooling system be linked to the extruder dye end to minimize diameter deformities. Organoleptic features, size, rheological properties, tensile strength, thermal behavior, and drug content are all examples of routine quality control testing. Dissolution equipment or Franz diffusion cells should also be used to determine drug release.22
The Healthcare Market For 3D Printing is Estimated to Expand
In 2020, the worldwide 3DP for healthcare market was worth US $8.087B, and it is expected to expand at a CAGR of 17.5% from 2021 to 2031. One of the major drivers in the worldwide 3DP in healthcare market is the increasing usage of this technology in various applications such as transplantation, medication delivery, 3D printed organs, and many more in the healthcare sector.23 Increasing need for implant modifications during surgical operations, as well as rising R&D expenditures, are driving the worldwide 3DP healthcare market. Due to widespread use of this manufacturing technology, improvements in it, and significant R&D expenditures, the North American market for 3DP solutions produced the most revenue between 2013 and 2017. In 2017, the United States had the largest revenue share in the regional market, owing to the growing need for rapid prototyping from a variety of industries. Furthermore, the growing number of government Research and Development (R&D) efforts in this manufacturing technique promotes regional market development. Asia-Pacific (APAC) will expand at the highest rate in the future years, owing to lower final product manufacturing costs, increased demand for customized goods, and more R&D expenditures, particularly in India, Japan, and China.24
Conclusion
FDM 3DP is a versatile technique that has been extensively researched for the manufacture of a variety of medication delivery devices. Global research organizations are presently trying to discover the intricacies of the manufacturing process, and despite the significant progress achieved so far, tangible planning is required to bring these goods to reality. The opportunity for 3DP to create customized medicinal products is obvious; nevertheless, machine modifications are required for appropriate pharmaceutical usage. Furthermore, a feasible manufacturing method requires the involvement of the pharmaceutical sector (to extrude filaments on a large scale) and internet pharmacies (to print drugs according to patient-specific prescriptions). Finally, regulatory and patent authorities should collaborate with businesses to provide a clear route to market.
References
- What is Rapid Prototyping? - Definition, Methods and Advantages. Twi-global.com. https://www.twi-global.com/technical-knowledge/faqs/faq-manufacturing-what-israpid-prototyping. Published 2021. Accessed August 30, 2021.
- Manda V, Kampurath V, Msrk C. 3D Printing and its Effect on Outsourcing: A Study of the Indian Aircraft Industry. J Aerosp Technol Manag. 2018;10. doi:10.5028/jatm.v10.862
- Diment L, Thompson M, Bergmann J. Clinical efficacy and effectiveness of 3D printing: a systematic review. BMJ Open. 2017;7(12):e016891. doi:10.1136/bmjopen-2017-016891
- Tofail S, Koumoulos E, Bandyopadhyay A, Bose S, O’Donoghue L, Charitidis C. Additive manufacturing: scientific and technological challenges, market uptake and opportunities. Mater Today. 2018;21(1):22-37. doi:10.1016/j.mattod.2017.07.001
- Nadagouda M, Rastogi V, Ginn M. A review on 3D printing techniques for medical applications. Curr Opin Chem Eng. 2020;28:152-157. doi:10.1016/j.coche.2020.05.007
- Tofail S, Koumoulos E, Bandyopadhyay A, Bose S, O’Donoghue L, Charitidis C. Additive manufacturing: scientific and technological challenges, market uptake and opportunities. Mater Today. 2018;21(1):22-37. doi:10.1016/j.mattod.2017.07.001
- Fisher T. Welcome to the Third Industrial Revolution: The Mass-Customisation of Architecture, Practice and Education. Archit Des. 2015;85(4):40-45. doi:10.1002/ad.1923
- Tan D, Maniruzzaman M, Nokhodchi A. Advanced Pharmaceutical Applications of Hot-Melt Extrusion Coupled with Fused Deposition Modelling (FDM) 3D Printing for Personalised Drug Delivery. Pharmaceutics. 2018;10(4):203.
- Martin N, Domínguez-Robles J, Stewart S, et al. Fused deposition modelling for the development of drug loaded cardiovascular prosthesis. Int J Pharm. 2021;595:120243. doi:10.1016/j.ijpharm.2021.120243
- Won J, Kim J, Gao G, et al. 3D printing of drug-loaded multi-shell rods for local delivery of bevacizumab and dexamethasone: A synergetic therapy for retinal vascular diseases. Acta Biomater. 2020;116:174-185. doi:10.1016/j.actbio.2020.09.015
- Eleftheriadis G, Fatouros D. Haptic Evaluation of 3D-printed Braille-encoded Intraoral Films. Eur J Pharm Sci. 2021;157:105605. doi:10.1016/j.ejps.2020.105605
- Goyanes A, Buanz A, Hatton G, Gaisford S, Basit A. 3D printing of modified-release aminosalicylate (4-ASA and 5-ASA) tablets. Eur J Pharm Biopharm. 2015;89:157-162.doi:10.1016/j.ejpb.2014.12.003
- Kempin W, Domsta V, Grathoff G, et al. Immediate Release 3D-Printed Tablets Produced Via Fused Deposition Modeling of a Thermo-Sensitive Drug. Pharm Res. 2018;35(6). doi:10.1007/s11095-018-2405-6
- Arafat B, Wojsz M, Isreb A, et al. Tablet fragmentation without a disintegrant: A novel design approach for accelerating disintegration and drug release from 3D printed cellulosic tablets. Eur J Pharm Sci. 2018;118:191-199. doi:10.1016/j.ejps.2018.03.019
- Scoutaris N, Ross S, Douroumis D. 3D Printed “Starmix” Drug Loaded Dosage Forms for Paediatric Applications. Pharm Res. 2018;35(2):34. doi:10.1007/s11095-017-2284-2
- Kollamaram G, Croker D, Walker G, Goyanes A, Basit A, Gaisford S. Low temperature fused deposition modeling (FDM) 3D printing of thermolabile drugs. Int J Pharm. 2018;545(1-2):144-152. doi:10.1016/j.ijpharm.2018.04.055
- Melocchi A, Parietti F, Loreti G, Maroni A, Gazzaniga A, Zema L. 3D printing by fused deposition modeling (FDM) of a swellable/erodible capsular device for oral pulsatile release of drugs. J Drug Deliv Sci Technol. 2015;30:360-367. doi:10.1016/j.jddst.2015.07.016
- Goyanes A, Wang J, Buanz A, et al. 3D Printing of Medicines: Engineering Novel Oral Devices with Unique Design and Drug Release Characteristics. Mol Pharm. 2015;12(11):4077- 4084. doi:10.1021/acs.molpharmaceut.5b00510
- Korte C, Quodbach J. Formulation development and process analysis of drug-loaded filaments manufactured via hot-melt extrusion for 3D-printing of medicines. Pharm Dev Technol. 2018;23(10):1117-1127. doi:10.1080/10837450.2018.1433208
- Gioumouxouzis C, Katsamenis O, Bouropoulos N, Fatouros D. 3D printed oral solid dosage forms containing hydrochlorothiazide for controlled drug delivery. J Drug Deliv Sci Technol. 2017;40:164-171. doi:10.1016/j.jddst.2017.06.008
- Melocchi A, Parietti F, Loreti G, Maroni A, Gazzaniga A, Zema L. 3D printing by fused deposition modeling (FDM) of a swellable/erodible capsular device for oral pulsatile release of drugs. J Drug Deliv Sci Technol. 2015;30:360-367. doi:10.1016/j.jddst.2015.07.016
- Verstraete G, Samaro A, Grymonpré W, et al. 3D printing of high drug loaded dosage forms using thermoplastic polyurethanes. Int J Pharm. 2018;536(1):318-325. doi:10.1016/j. ijpharm.2017.12.002
- https://finance.yahoo.com/news/global-pharmaceutical-packaging-materialsmarket-143000313.html
- https://www.psmarketresearch.com/market-analysis/3d-printing-market
About the Authors
Prachi Khamkar obtained her Bachlors degree from Shivaji University, Kolhapur in Pharmaceutical Science. She is currently working as Project Head, Pharmaceutical Manufacturing Operations, CiREE, Pune. At CiREE she has guided students with pharmaceutical backgrounds from various universities across India. She also works as a scientific content writer at AM Chronicle- an information and content platform for additive manufacturing and 3D printing industry based in Mumbai. Her area of interest includes pharmaceuticals 3D printing and topical and transdermal drug delivery.
Debarshi Kar Mahapatra has published research review papers in reputable international journals. He has also contributed book chapters, opinions, magazine articles; authored many textbooks; and has edited a few books. He has presented his research at several international platforms for which he received awards from numerous professional bodies. He has been recognized as one of the Top 2% International Scientists by Stanford University. Presently, he is serving as a reviewer and editorial board member for international journals.
Subscribe to our e-Newsletters
Stay up to date with the latest news, articles, and events. Plus, get special offers
from American Pharmaceutical Review – all delivered right to your inbox! Sign up now!