Robert Dream- Managing Director, HDR Company LLC
End-to-End (from seed to unit dose - E-2-E) manufacturing represents the next generation of biopharmaceutical manufacturing processes for both large and small molecules. It is recognized by regulatory authorities as a key emerging technology. The FDA has approved various small molecule products and recently issued draft guidance for industry on continuous manufacturing. The International Council for Harmonization issued (ICH) Q135 which has been adopted by the European Medicines Agency (EMA) to support the technology and give guidance as well. Companies can add flexibility and maximize the value of process analytics ICH Q146 to enhance E-2-E manufacturing.
It is important to note that regulatory guidance on process analytical technology (PAT) includes ICH Q8(R2),8 ICH Q2(R2),7 Figure 1, and FDA’s “Guidance for Industry” from 2004.9
The FDA PAT guidance considers process analytical technology to be:
“A system for designing, analyzing, and controlling manufacturing through timely measurements (i.e., during processing) of critical quality and performance attributes of raw and in-process materials and processes, with the goal of ensuring final product quality. It is important to note that the term analytical in process analytical technology is viewed broadly to include chemical, physical, microbiological, mathematical, and risk analysis conducted in an integrated manner.”
E-2-E manufacturing analytics must move closer to the process. Process analytical technology can therefore add significant value in design, analysis, and control of the E-2-E manufacturing process. Process analytical technology does not involve pooling or holding of the process, and decisions could be made in Real Time Release Testing (RTRT).4 e.g., when designing equipment or modeling unit operations, process analytical technology measurements used to determine Residence Time Distribution (RTD) are critical. During process development, process analytical technology may be used to confirm satisfactory process operation, verify models, inform on divert to waste (DTW) situations, or perform feedback/feedforward controls. The control strategy for a E-2-E process may also benefit from the use of process analytical technology. Furthermore, process analytical technology can be used for final critical quality attribute (CQA) determination directly or as input to a more complex model that includes critical process parameters (CPP) and critical material attributes (CMA).
CQA = f[CPP1 +CPP2 +CPP3 +…, CMA1 +CMA2 +CMA3 +…]
When developing and implementing a commercial E-2-E manufacturing line, process analytical technology is recommended, even more strongly than it is for batch manufacturing, for all three phases: design, analysis, and control. The extent of process analytical technology use is company and product-dependent, reflecting the perceived return on investment.
Continuous manufacturing has several advantages over batch operation in terms of process yield, process efficiency, facility utilization, and process consistency. These advantages offer several opportunities for the next generation of biomanufacturing facilities with respect to their design, construction, and operation.
- Opportunities in implementing continuous biomanufacturing
- Higher process yield
- Higher process efficiency
- Flexible facilities » Stable production
- Improved product quality
- Process consistency
- Flexibility to respond to manufacturing needs
- Decreased need for comparability
- Reduced manufacturing footprint
- Single-Use technology
- Reduced media volumes
- Simpler cleaning
- Better product understanding
- Inline/online monitoring and control (RTRT)
- Equipment could withstand long runs
- Digital Sensors that could accommodate RTRT
- Experienced workforce (could manage E-2-E manufacturing)
- Challenges for implementing continuous biomanufacturing
- Process complexity
- Cell retention
- High cell density
- Mixing
- Aeration
- Process control
- Longer run times
- Process reliability and stability
- Cell line stability
- Maintaining sterility
- Process scalability (in a continuous perfusion process)
- Process scale-up
- Process consistency and control
- Process characterization
- Complexity of scale-down model (for perfusion process)
- Process optimization and characterization (for a perfusion process)
- Process validation
- Raw material properties and variability
- Impurities and removal (degradation of product over time)
- Viral safety and bioburden
- Material traceability
- Cell line stability and life span
- Product knowledge and structural/ functional relationship
- Analytical technology and control strategy
- Design spaces and potential interactions between steps
- Evaluation of manufacturing changes & impact on product quality
Biomanufacturing is an established industry generating valuable medicines for the treatment of life-threatening diseases including cancer, autoimmune diseases, and metabolic and blood diseases.2,3 Therapeutic proteins such as interferons, blood clotting factors, enzymes, vaccines, and monoclonal antibodies (mAbs) are produced from genetically engineered microbial, yeast, and mammalian cells using validated processes involving several unit operations and employing characterized and optimized process parameters.1,2
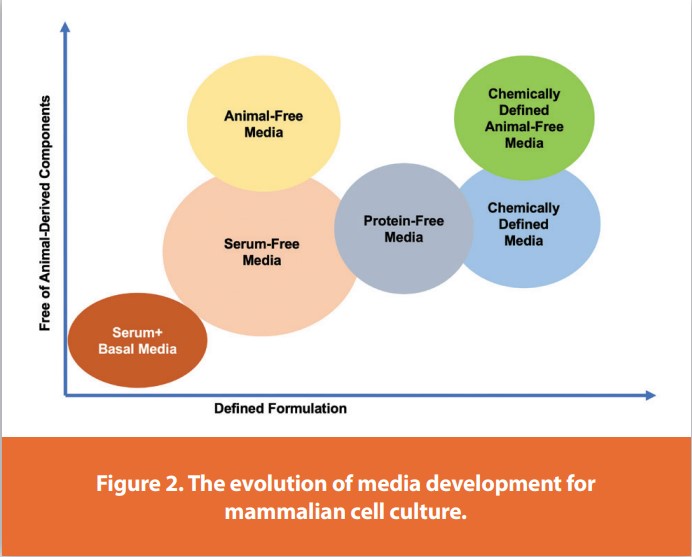
Since the early days of biomanufacturing process development there has been a great interest in continuous processing.10,11 Continuous culture with cell retention, and perfusion, was very popular in the 1980s as it allowed reaching high cell densities and thus higher volumetric productivities. The cell culture media, Figure 2; used in those days were not very rich in nutrients and as a result cell densities achievable in batch mode were about 1-2 million ml-1. Continuous perfusion operation allowed an order of magnitude increase in the cell density in the bioreactor by perfusing medium at an exchange rate of 2-10 bioreactor volumes a day. Continuous perfusion also offered better quality for usable, labile proteins, such as enzymes and blood coagulation factors.10-13
Higher Process Yields
One of the main advantages of continuous biomanufacturing over batch manufacturing is achieving a higher productivity per volume. A comparison of a batch and continuous process is presented in Table 1, for mAb production, Figure 3.
Higher Process Efficiency
A batch process is run for a short time and a lot of time is spent between batches for termination, cleaning, reconditioning of equipment, and preparation and build-up of the process intermediates for the next batch (downtime) impacts the cycle time and plant utilization efficiency. Continuous processes can be operated for a long time minimizing the impact of the downtime on the overall utilization rate, Figures 4 and 5.
Improved Product Quality
Continuous processing offers opportunities in biomanufacturing by increasing the speed and reducing and or eliminating the hold time, or residence time, during the process. Reducing the residence time during the cell culture process is highly beneficial to minimizing product degradation for labile protein products.
Flexible Facilities
A flexible factory is a production facility that can rapidly reconfigure to produce different drug products. A flexible factory can change what it produces at relatively short notice. Hence, the word ‘flexible’ in the term. The creators of the flexible factory deliberately designed it so that it can provide a wide range of operations and services to produce drug substances and drug products.
Using an end-to-end set-up that’s designed to produce certain drug product within a family of drug products. The line(s) will have interchangeable equipment/systems that could be reconfigured, Figure 6.
Scalable Production
E-2-E or continuous production is a flow production method used to manufacture, produce, or process materials without interruption. Continuous production is called a continuous process or a continuous flow process, because the materials, either dry bulk or fluids that are being processed are continuously in motion. Continuous usually means operating 24 hours per day, seven days per week with infrequent maintenance shutdowns.
Scaling-up such a process is more involved than scaling-up a batch process. Usually, continuous manufacturing has a set equipment and systems size and in case of the need for production increase the process is scaled-out. By scaling-out the process the need for additional product is accomplished and simplifies the validation and approval process.
Risk Mitigation and Risk Management
Risk mitigation and process debottlenecking with in-time delivery/release and E-data integration is essential to enable continuous biomanufacturing.
Higher titers in monoclonal antibody processes and newer cell and gene therapy products have introduced various new technologies into the biopharma market. While these new technologies have furthered the field as a whole, they have also generated several new challenges in raw material management from a regulatory, quality and supply chain standpoint. The manufacturing process innovation that leads to increased flexibility while decreasing overall drug product cost can help meet some of the market challenges. A lot of progress has been made to improve upstream to downstream unit operations.
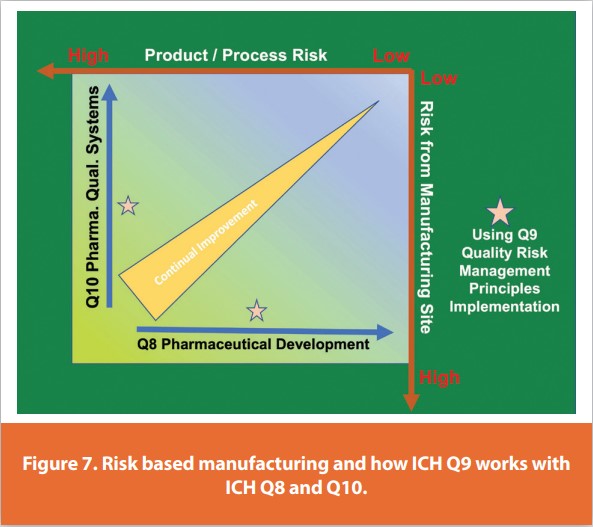
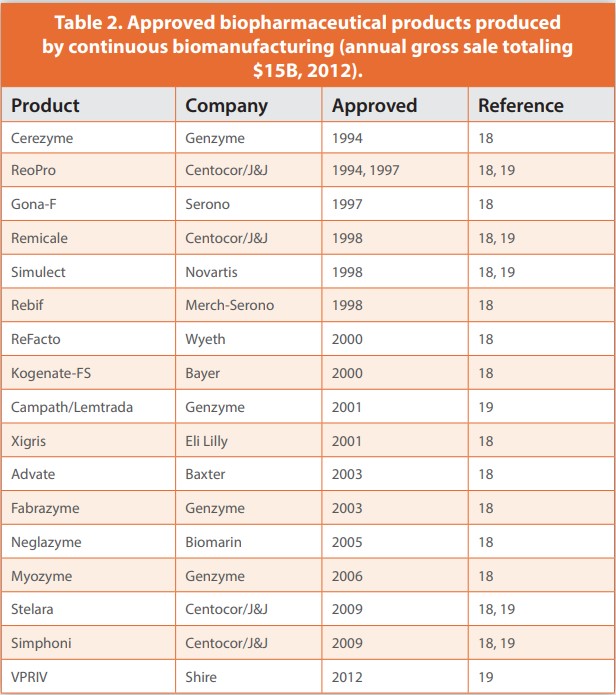
However, many challenges need to be overcome to implement well-functioning fully integrated continuous manufacturing. Typical biologic manufacturing consumes hundreds of raw materials including media, supplements, process chemicals, filters, resins, and excipients. Managing the flow of these components and releasing them on a timely basis is a daunting task without either increasing inventory levels or implementing a non-traditional approach to receive release. Using these materials while meeting all quality and regulatory requirements and predicting process variations are key to de-risking the manufacturing process. In general, using ICH Q9, Q8 and Q10 to eliminate and or reduce the risk and maintain the safety, efficacy, and quality of the drug substance-drug product is recommended (Figure 7).
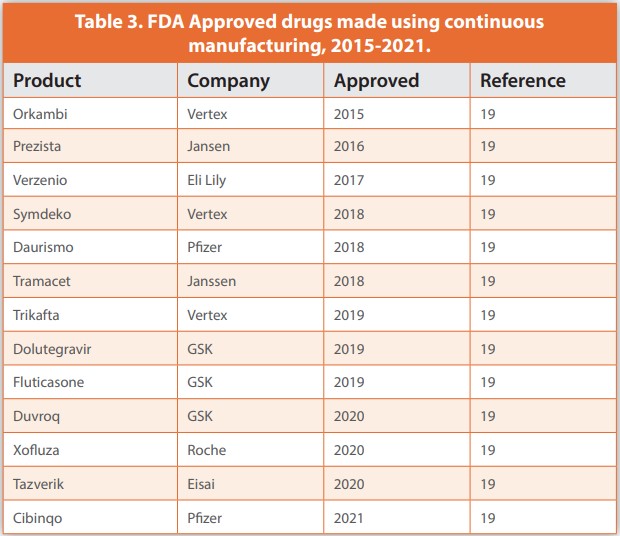
Although several products have been developed using continuous cell culture process, the complexity of running a perfusion operation, and ensuring its reliability, scalability, and manufacturability have hampered its wide spread acceptance by the pharmaceutical industry.3,14,18 By the early 1990s batch operation emerged as the method of choice for biomanufacturing.15,16,17 Three factors played a role in the move:
1. Simplicity of batch operation
2. Focus on monoclonal antibodies by pharmaceutical companies
3. Advancement in feed development and medium enrichment, Figure 2
Monoclonal antibodies are relatively robust proteins and their quality is not impacted by residence time. By using an optimized feed strategy in a fed-batch with specialty feed solution and medium, more than a one order of magnitude increase in cell density and in productivity was accomplished. All of these factors combined established the fed-batch operations as the platform of choice and continuous processing remained an option for special molecules only.2,17
Continuous processing is an important part of biomanufacturing as evident from the number of products in the market currently at more than 90 (Table 2 and 3). There are about 30+ biological products ranging from replacement enzymes, blood coagulation factors, and monoclonal antibodies. Some of these products generate more than a billion dollars a year with Remicade from J&J reaching about US$6.8B in sales in 2012.
While platform technologies are commonly used fed-batch is the platform of choice, enhancing productivity and reducing manufacturing cost prompting companies to consider alternate manufacturing technologies. Continuous processing offers a number of advantages over batch and it has been successfully implemented in other industries. Use of continuous processing in biomanufacturing started with culture as discussed and it is feasible to apply it to all manufacturing unit operations in the future. Many of the issues related to the implementation of continuous processing have been resolved due to the recent advances in bioprocess engineering, automation, and process optimization.
Advantages and Disadvantages of Continuous Biomanufacturing
The multifaceted advantages of continuous biopharmaceutical manufacturing are summarized in Table 4. A model comparison of continuous bioprocessing platform with stainless steel and single-use batch processes across clinical and commercial scales suggests that continuous operation can boost the savings afforded by single-use technologies.23 The reduction in long-term production costs can make the pursuit of biosimilars economically more attractive for drug companies.
In addition to affordability, continuous manufacturing can also improve the accessibility of drugs.24 Failure in manufacturing quality can lead to drug shortages,24,25 and continuous manufacturing can deliver products of consistent quality. The ability to produce (plug and produce, or plug and play) potentially higher-quality drugs on demand can circumvent overproduction and delays.26
A comparison of batch versus continuous manufacturing processes is illustrated in Table 4.
The Drive to Innovation and Industry Excellence
The past decade has seen a significant shift in the nature of the products being manufactured and sold by the innovative biopharmaceutical industry. The global biopharmaceutical portfolio of today reflects increased therapeutic competition, a greater prevalence of large molecule drugs, advanced therapy medicinal products, expansion in the number of personalized or targeted products, and a rise of treatments for many orphan and rare diseases. These next generation trends, Figure 8, have given rise to biopharmaceutical products with extremely limited production runs, highly specific manufacturing requirements, and genotype-specific products.
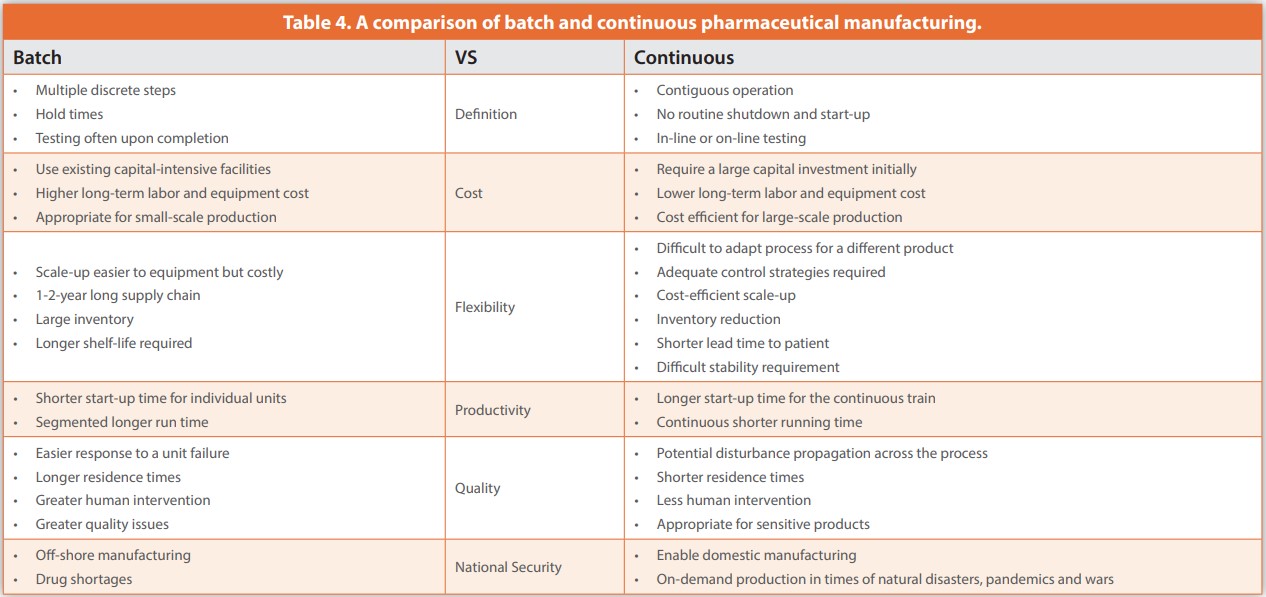
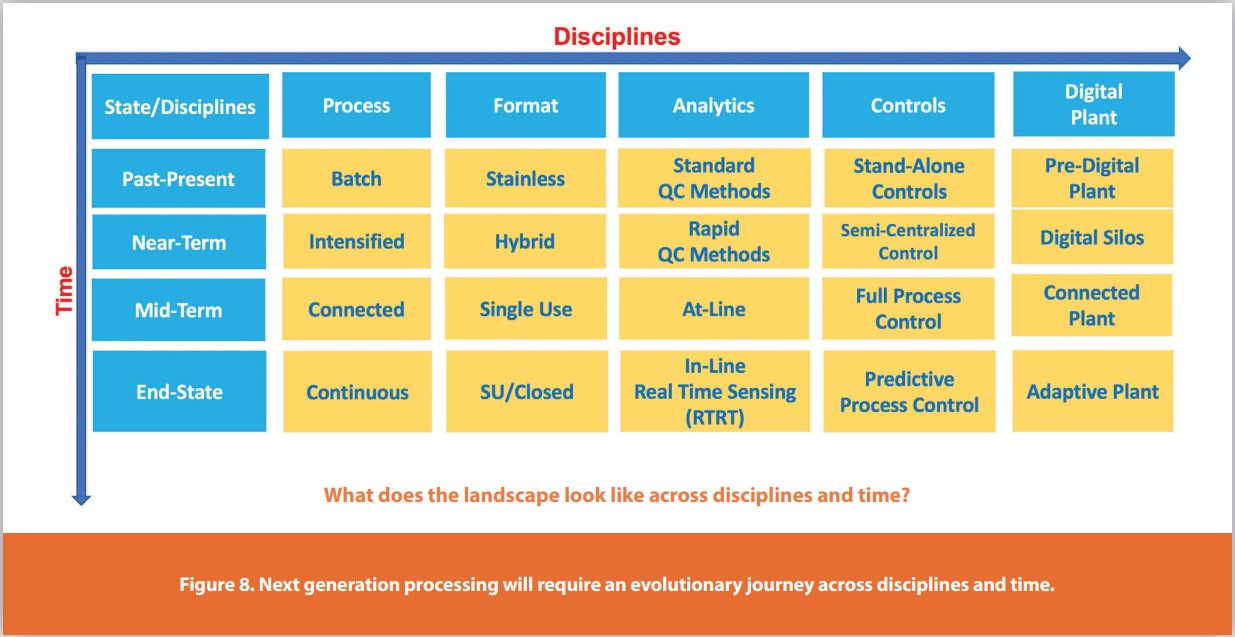
This fundamental shift in the overall product mix and a focus on continuing to improve the efficiency and effectiveness of production is spurring an evolution in the technologies and processes needed to support advanced biopharmaceutical manufacturing. Innovation in manufacturing technology is helping to drive improved economics, flexibility and quality while potentially benefiting patients both directly and indirectly. Biopharmaceutical manufacturers are generally making investments in the following areas:
- Continuous manufacturing to improve scalability and facilitate time to market, while lowering capital and operating costs and enhancing quality
- New process analytical tools to improve process robustness, accelerate scale-up to commercial production and drive more efficient use of resources
- Single-use systems to increase flexibility and reduce production lead times, while lowering capital investment and energy requirements
- Alternative downstream processing techniques to improve yields while lowering costs, green chemistry to reduce waste, and new vaccine and therapy production methods to increase capacity, scalability, and flexibility
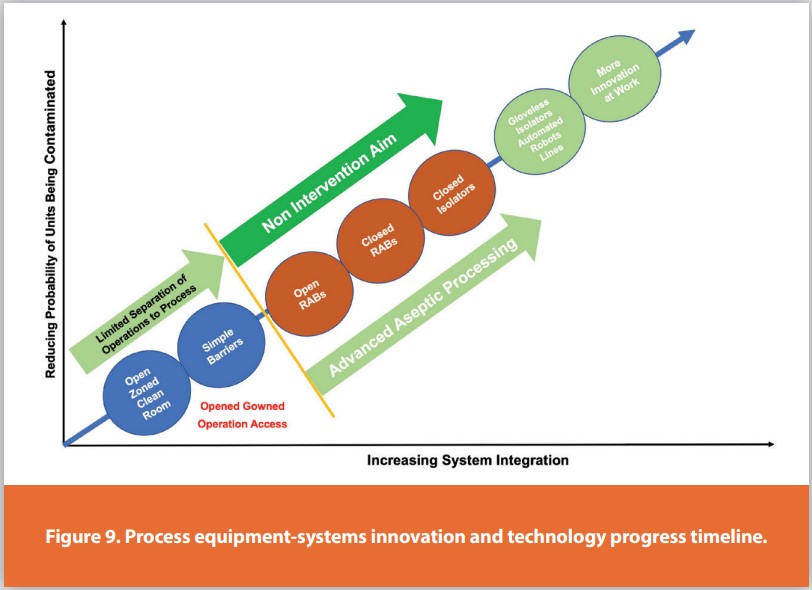
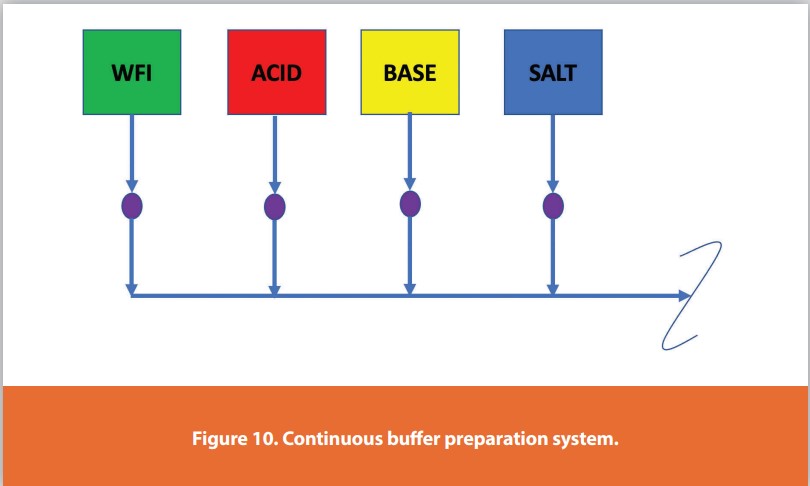
Additionally, new types of products are coming to market that help increase the effectiveness of medicines and support patient compliance, such as products that reflect improvements in drug delivery systems and drug-device combination products. These products require advanced manufacturing techniques on the part of the biopharmaceutical company and its supply network, as the manufacturing process itself is becoming more central to the effectiveness of medicine. The changes in biopharmaceutical portfolios and the rise of advanced manufacturing technologies have impacted both inside and outside of biopharma companies. As a result, the suppliers of automation, equipment design, sensor technology, and facilities design have stepped up to support the drug biomanufacturing space to match the innovation in medicine as well, Figure 9.
Advances in Buffer Preparation
Advances in buffer manufacturing and preparation, whether it would be saving space, saving time or saving cost, the system is coming up to support continuous manufacturing, Figure 10.
The connected buff er management system delivers the buffers needed using in-line dilution at the point of use to free the process floor space and streamline the workflow and eliminate the buff er preparation processes suite requirement.
The flexible, automated system supports buffer needs from pilot scale to clinical scale production up to 1200 lhr-1 with up to six different formulations delivered within the target specifications from up to 20x concentrates.
Hybrid Continuous (Semi-Continuous) Bioprocessing (HCB) Platform
Actual use of perfusion for commercial manufacture is more widespread, but bioprocessing manufacturers are becoming increasingly reticent to publicly share details about their processes. To point out, manufacturers such as Genzyme, Bayer, Janssen, Merck-Serono, Novartis, and Lonza for Eli Lilly manufacture approximately 19 marketed recombinant protein and monoclonal antibody (mAb) products using perfusion or elements of continuous processing, and these products are predominantly blockbusters with annual revenues totaling ~$20 billion.20
References
- Kelly, B. 92009) Industrialization of mAb production technology. The bioprocess industry at a crossroad. mAbs, I, 443-452.
- Shukla, A.A. and Thömmes, J. (2012) Recent advances in Large-Molecule production of monoclonal antibodies and related proteins, Trends Biotechnol., 28 (5), 253-261.
- Ozturk, S.S. and Hu, W.S. (eds) (2006) Cell culture technology for pharmaceutical and Cell-Based Therapies, CRC Press, Taylor & Francis Group, Boca Raton, FL.
- Guideline on Real Time Release Testing (formerly Guideline on Parametric Release). Real Time Release Testing guideline (europa.eu)
- Q13 Continuous Manufacturing of Drug Substances and Drug Products, March 2023; Q13 Continuous Manufacturing of Drug Substances and Drug Products (fda.gov)
- ICH Harmonised Guideline, Q14 Analytical Procedure Development, Step 2, 08/26/2022; Q14 Analytical Procedure Development (fda.gov)
- ICH Harmonized Guideline, Validation of Analytical Procedures Q2(R2), March, 24 2022; ICH_Q2-R2_Document_Step2_Guideline_2022_0324.pdf
- ICH Harmonized Tripartite Guideline Pharmaceutical Development Q8(R2), August 2009; Q8(R2) Guideline.pdf (ich.org)
- Guidance for Industry PAT — A Framework for Innovative Pharmaceutical Development, Manufacturing, and Quality Assurance, September 2004; Guidance for Industry PAT - A Framework for Innovative Pharmaceutical Development, manufacturing, and Quality Assurance (fda.gov)
- Bonham-Carter, J. and Shevitz, J. 920110 A brief history of perfusion biomanufacturing how high. Concentration cultures will characterize the factory of the future. BioProcess Int., 9(9), 24-31.
- Ozturk, S.S. (2013) Cell culture technology; a historical perspective with future directions. Keynote lecture. annual biomanufacturing Leaders Summit, Boston, MA, pp. 11-12.
- Warikoo, V., Godawat, R., Brower, K., Jain, S., Cummings, D., Simons, E., Johnson, T., Walther, J., Yu, M., Wright, B., McLarty, K.P. Hwang, C., Zhou, W., Riske, F., and Konstantinov, K., (2012) Integrated Continuous Production of recombinant therapeutic proteins. Biotechnol. Bioeng., 109 (12), 3018-3029.
- Vogel, J.H., Nguyen, H., Giovannini, R., Ignowski, J., Garger, S., Salgotra, A., and Tom, J. (2012) A new large-scale manufacturing platform for complex biopharmaceuticals. Biotechnol. Bioeng., 109 (12), 3049-3058.
- Pollack, J., Ho, S. V., and Farid, S.S. (2013) Fed-Batch and Perfusion culture Processes: economic, environmental, and operational feasibility under uncertainty. Biotechnol. Bioeng., 110 (1), 206-219.
- Jayapal, K.P., Wlaschin, K.F., Hu, W.-S., and Yep, M.G.S. (2007) Recombinant protein therapeutics from CHO cells-20 years and counting. Chem. Eng. Prog., 103, 40-47.
- Li, F., Vijayasankaran, N., Shen, a., Kiss, R., and Amannullah, A. (2010) Cell culture processes for monoclonal antibody production, mAbs, 2 (5), 466-477.
- Andersen, D.C. and Reilly, D.E. (2004) Production technologies for monoclonal antibodies and their fragments. Curr. Open. Biotechnol., 15, 456-462.
- Ozturk, S.S. and Kompala, D., (2006) Optimization of high cell density perfusion columns, in Cell Culture Technology for Pharmaceutical and Cell-Based Therapies (eds S.S. Ozturk and W.-S. Hu), Boca Raton, FL. Pp. 387-416.
- Developments and opportunities in continuous biopharmaceutical manufacturing, Ohnmar Khanal & Abraham M. Lenhoff, Article: 1903664, Received 04 Dec 2020, accepted 11 Mar 2021, Published online: 11 Apr 2021. Full article: Developments and opportunities in continuous biopharmaceutical manufacturing (tandfonline.com)
- 11th Annual Report and Survey of Biopharmaceutical Manufacturing, E. Langer, BioPlan Associates, April 2014, pp. 103-116.
- Ozturk, S.S. (2013) Cell culture technology: a historical perspective with future directions. Keynote lecture, Annual Biomanufacturing Leaders Summit.
- Dream, Robert, Continuous Manufacturing Progress and the Bio/Pharmaceutical Industry “Reality or Fad”, American Pharmaceutical Review, Tuesday, August 15, 2017.
- Hummel J, Pagkaliwangan M, Gjoka X, Davidovits T, Stock R, Ransohoff T, Gantier R, Schofield M, Modeling the downstream processing of monoclonal antibodies reveals cost advantages for continuous methods for a broad range of manufacturing scales. Biotechnol J. 2019; 14(2): 17000665. Doi:10.1002/biot.201.201700665.
- FDA, Drug shortage: root cause and potential solutions [internet]. 2019 [cited 2020 Apr 7]:1-24. Available from: https://www.fda.gov/news-events/press-announcement/ statement-fda-new-report-regarding-root-caus-and-potential-solutions-drug-shortages
- Fisher AC, Kamga MH, Agarabi C, Bronson K, Lee SL, Yoon S. the current scientific and regulatory landscape in advancing integrated continuous biopharmaceutical manufacturing, Trends Biotechnol. 2019;37(3):253-67. Doi: 10.1016/j.tibtech.2018.08.008.
- Srai JS, Badman C, Krumme M, Futran M, Johnson C, Future supply chains enabled by continuous processing-opportunities and challenges. Many 20-21, 2014, continuous manufacturing symposium. J. Pharm Sci. 2015;104(3):840-49. Dot:10.1002/jps.24343.
- Konstantin Konstantinov, VP, Late-Stage Process Development, Bio-Realization, Sanofi, (noted figures, via e-mal). 12/2016.
Subscribe to our e-Newsletters
Stay up to date with the latest news, articles, and events. Plus, get special
offers from American Pharmaceutical Review delivered to your inbox!
Sign up now!