Anis H. Khimani, PhD- Senior Strategy Leader, Life Sciences Strategy Group, Revvity; Tanaya Surve- Senior Scientist, Bioproduction Group, Revvity
Biotherapeutics - A Snapshot
Drug development has evolved significantly over the past couple of decades, particularly within the large molecule biotherapeutics arena. In a growing market, the therapeutic specificity of biotherapeutics has resulted in a number of modality options. In addition to offering precise targeting capabilities compared to traditional small molecule drugs, large molecule medications can potentially offer more profitable financial return. Drawing from the success of biotherapeutics, biosimilars are being developed as cost-effective alternatives to the high price point of first-generation drugs.
For the discovery and development of large molecule drugs, technologies have focused on advancing upstream and downstream stages of the value chain. Focus on critical factors upstream in drug design, enables companies to predict and prevent downstream issues that might lead to delays in drug approval or costly recalls. The importance of biotherapeutics continues to expand across various types of modalities from monoclonal antibodies, and with increasing interest in bispecific and multispecific antibodies, to nanobodies and recombinant proteins. Each modality offers several prophylactic or treatment options against disorders such as rheumatologic diseases, cancer, diabetes, anemia, infectious diseases, and more.
Cell Line Development in Biotherapeutics - A Convoluted Journey
The upstream workflows in biotherapeutics development have witnessed significant progress in cell line development (CLD), clone selection, scale-up, automation, and digitization to enable precision as well as productivity. These transformative advancements across the biotherapeutics value chain have also contributed to increased agility and enablement of regulatory compliance. The recent pandemic has accelerated the process to deliver drugs to market while still maintaining high quality, safety, and efficacy standards. From the COVID-19 experience, as scientists and innovators, we have learned that vaccines, small and large molecules, as well as related biotherapeutics development has called for more collaboration among drug developers, technology providers, supply chain organizations, and regulators at a global level.
Chinese Hamster Ovary (CHO) cell lines are widely used for the production of therapeutic proteins, and recent advances in engineering of CHO cells via next generation technologies such as gene editing have enabled knock-out cell lines with enhanced characteristics. For example, engineering of CHO cells for various metabolic pathways has been shown to improve final protein product quality and enhance expression levels.1,2 Genome editing systems using CRISPR/Cas9 have been powerful in understanding gene functions via modulation or knock-out, knock-in, or integrating gene-of-interest (GOI) into specific sites of the genome following the double-strand break (DSB) mediated by the CRISPR system.
Within the upstream stages of large molecule manufacture, CLD involves a long, time-consuming process and continues to be challenging due to clone instability and acceptable expression levels of the protein of interest.
Product variability can be introduced at various stages of the CLD process and includes, but is not limited to, variability in transfection, randomness of genetic integration in host cells that can lead to clonal heterogeneity, and instability and/or silencing of the transgene(s). Consequently, stable clonal cell banks are used for GMP production to minimize the variability of the final product. However, with the current race to clinic, more groups are striving to achieve homogeneity in product quality earlier in the process, hence enabling pre-clinical studies using material produced at early stage of the CLD (i.e., pools).3-5
Transposon-Based Technologies
To overcome the above limitations, genetic elements called transposons have been used as vehicles to deliver a GOI into the host genome. Transposon systems (e.g., Sleeping Beauty, Leap-in, PiggyBac and Tol2) preferentially integrate a GOI into active transcriptional sites,6 allowing rapid cell line development, and resulting in high titers of the recombinant protein. Transposons are therefore an ideal vehicle for transporting a GOI into genomes.7
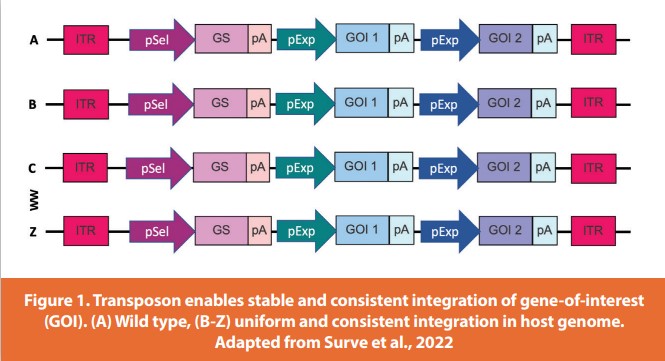
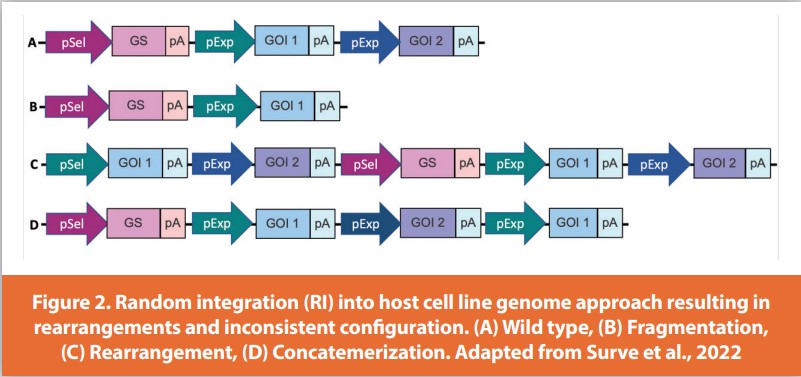
DNA transposons are comprised of transposase gene and inverted terminal repeat (ITR) sequences. The activity of transposase and insertion of transposon fragments into the genomic site occurs via a “cut-and-paste” mechanism.8 The basic vector design for using transposons to deliver recombinant genes consists of the GOI and selection marker flanked by the ITRs, and a transposase gene located on a separate vector in trans. Nevertheless, to control the activity of the transposase gene, and hence the mobility of the gene cargo, the transposase is often provided in the form of mRNA. The use of transposon-based vectors virtually eliminates risks of integration of bacterial elements present on the transposon vector backbone, since the integration of the transgene is catalyzed by the transposase that specifically recognizes the ITRs flanking the GOI and the selection cassette. Furthermore, this expression system improves reproducibility between different transfections, and therefore improves clonal stability.9
Transposon-based technologies along with established CHO cell lines have been adopted within CLD and large molecule expression workflows. Transposon-based expression systems enable multi-copy, non-fragmented gene cassette integration.10 This transposon approach results in clonal stability of >95%, at both genetic and phenotypic level minimizing clone screening efforts, thus simplifying process development and streamlining scale up.11
This technology is generally a two-component system, a transposon vector, and a transposase preferably in the form of mRNA, both of which can be co-transfected into CHO cell lines. Upon translation of the mRNA, the resulting transposase catalyses the transgene excision and integration into the cell line genome. One advantage of the transposon system compared to random integration expression vectors, is that it allows intact integration of the expression cassette at each integration site (Figure 1) and is therefore devoid of any fragmentation (Figure 2B), or rearrangement (Figure 2C) or concatemerization (Figure 2D). As a result, directed integration of the GOI at transcriptionally active sites within the genome of the cell line leads to homogeneity and clonal stability of greater than 95%.
Summary – Future Insights
In the past couple of decades, large molecule drug development has advanced along both upstream and downstream stages in the context of streamlining processes, establishment of Critical Quality Attributes (CQAs), compliance requirements, as well as technologies that support the workflows. From a biological and technical perspective, upstream cell line development for the production of large drug molecules is a critical and founding step that not only contribute to the product titer yields and scale up, but also defines the technologies that will be required to eliminate cellular artifacts as well as the final purification of the drug.
As discussed above, the CHO cell line has been widely used for a long time for protein drug expression and production. Recent gene editing and GOI delivery vectors, and related mechanisms have enabled targeted and consistent cell line engineering.
Transposon technologies have offered specific launch pads to simplify development and reduce manufacturing effort. Additional advancement and standardization of cell counting and clone selection methods via automated cell evaluation technologies will further streamline the end-to-end workflow during CLD. Integration of processes and protocols with automation, detection and cell imaging technologies, as well as data analysis and management will redefine the process to scale upstream cell line development. Machine learning will further facilitate advanced predictive and data models to establish best practices and eliminate process gaps. A combinatorial and collaborative approach across various functional and process areas will lead to next-generational biologics models for precision, reliability, and efficacy.
References
- Mistry, R.K., Kelsall, E., Sou, S.N., Barker, H., Jenns, M., Willis, K., Zurlo, F., Hatton, D., and Gibson, S., 2021. A novel hydrogen peroxide evolved CHO host can improve the expression of difficult to express bispecific antibodies. Biotechnol. Bioeng., 118:2326.
- Budge, J.D., Knight, T.J., Povey, J., Roobol, J., Brown, I.R., Singh, G., Dean, A., Turner, S., Jaques, C.M., Young, R.J., Racher, A.J., and Smales, C.M., 2020. Engineering of Chinese hamster ovary cell lipid metabolism results in an expanded ER and enhanced recombinant biotherapeutic protein production. Metab. Eng. 57:203.
- Scarcelli JJ, Shang TQ, Iskra T, Allen MJ, Zhang L. Strategic deployment of CHO expression platforms to deliver Pfizer’s Monoclonal Antibody Portfolio. Biotechnol Prog. 2017 Nov;33(6):1463-1467. doi: 10.1002/btpr.2493. Epub 2017 Jun 2. PMID: 28480558.
- Stuible, M., Lier, F.V., Croughan, M.S., & Durocher, Y. (2018). Beyond preclinical research: production of CHO-derived biotherapeutics for toxicology and early-phase trials by transient gene expression or stable pools. Current Opinion in Chemical Engineering.
- Wright C, Alves C, Kshirsagar R, Pieracci J, Estes S. Leveraging a CHO cell line toolkit to accelerate biotherapeutics into the clinic. Biotechnol Prog. 2017 Nov;33(6):1468-1475. doi: 10.1002/btpr.2548. Epub 2017 Sep 7. PMID: 28842948.
- Wei, M., Mi, C-L., Jing, C-Q., and Wang, T-Y, 2022. Progress of transposon vector system for production of recombinant therapeutic proteins in mammalian cells.
- Sandoval-Villegas, N., Nurieva, W., Amberger, M., Ivics, Z., 2021. Contemporary transposon tools: A review and guide through mechanisms and applications of sleeping beauty, piggyBac and tol2 for genome engineering. Int. J. Mol. Sci. 22:5084.
- Matasci, M., Baldi, L., Hacker, D.L., and Wurm F.M., 2011. The piggyBac transposon enhances the frequency of CHO stable cell line generation and yields recombinant lines with superior productivity and stability. Biotechnol. Bioeng. 108:2141.
- Narayanavari, S.A., Izsvák, Z., 2017. Sleeping Beauty transposon vectors for therapeutic applications: advances and challenges. Cell Gene Therapy Insights 2017; 3(2), 131-158, 10.18609/cgti.2017.014.
- Patel, T., Ingham, C., Fleming, J., Surve, T., Cougot, D., Ashtley, H., and Zurdo, J., 2022. CHOSOURCE™ ADCC+ cell line for enhanced therapeutic potency. https://horizondiscovery. com/-/media/Files/Horizon/resources/Posters/chosource-chok1-adcc-cell-line-poster. pdf. Accessed on 23 May 2023
- Surve, T., Rebocho, A., Fleming, J., Pinto, N., Patel, T., Sandeman, K., Claudia, C., Cougot, D., Astley, H., and Zurdo, J., 2022. Integration of next-generation transposon vectors with novel host cell lines. https://horizondiscovery.com/ /media/Files/Horizon/resources/ Posters/chosource-tnt-poster.pdf. Accessed on 23 May 2023.
Author Biographies
Anis H. Khimani, PhD, is Senior Strategy Leader with the Life Sciences strategy group at Revvity. Anis has prior academic and research background in molecular biology, viral pathogenesis, vector development, and vaccine studies from Harvard Medical School and Dana-Farber Cancer Institute. After his academics and research, he pursued research and development in genomics and assay development, as well as strategy and product management in informatics at PerkinElmer. Subsequently, his focus has been in strategy and market segment development within Life Sciences.
Tanaya Surve is Senior Scientist with the Bioproduction group at Revvity. Tanaya has over 11 years of experience in cell line development and has contributed to the drug product development and launch of several molecules of therapeutic importance. Her current role focuses on vector development and cell line engineering to create improved host cell platforms. She holds a Master’s in Biotechnology and a diploma in International Business Management.
Subscribe to our e-Newsletters
Stay up to date with the latest news, articles, and events. Plus, get special
offers from American Pharmaceutical Review delivered to your inbox!
Sign up now!