Mark Rogers, PhD - Global Scientific Director, SGS Health & Nutrition
Messenger RNA (mRNA) technology was discovered in 1961, but it was not until the 1990’s that the concept of mRNA-encoded drugs was demonstrated and another several decades before the first mRNA product was approved by the USFDA.
The development of mRNA-based therapies has followed a long and somewhat unique historical path. Today, one such therapy, a COVID-19 vaccine, is one of the most widely used and globally recognized medicines. In 2023, Katalin Karikó and Drew Weissman were awarded the Nobel Prize in Physiology or Medicine, an acknowledgment of their work in the development of mRNA-based vaccines and a testament to their many years of resilience against some harsh skepticism.
The positive influence on mRNA therapeutic development resulting from the Covid pandemic cannot be overstated. However, the need for strong and detailed analytical examination of such products has also recently been highlighted. Some reporting suggests unexpected, delocalized biodistribution of mRNA and protein products after inoculation with the mRNA vaccine and DNA plasmid contamination has been observed in certain mRNA vaccines.1
Currently, there are three major applications for mRNA-based therapies: prophylactic vaccines, therapeutic vaccines and therapeutic drugs which have been comprehensively reviewed in a recent publication by Wang.2 The manufacture of such products ranges from the use of cellular systems to fully synthetic regimens.
Most conventional therapies work by binding and inhibiting hyperactive disease-causing proteins while mRNA therapies restore protein activities or, in the case of vaccines, may elicit new protein entities thereby reversing or obstructing the disease state. The specificity of this modality provides for low, off-target effects and minimal risk of causing genetic mutation since mRNA does not enter the cell nucleus.
To date, two main forms of mRNA vaccines have been developed: non- replicating and self-amplifying mRNA (SAM), the latter containing sequences that encode for replicases that direct intracellular mRNA amplification.
The development of mRNA as the basis of a therapeutic class has been largely challenged by the molecule’s somewhat poor stability, immunogenicity, and issues involving it’s in vivo delivery. However, while still being refined, some of these problems have been addressed by modifications to the mRNA sequence which may be segregated into four defined components: a 5’ end cap, upstream and downstream untranslated regions (UTR), a coding region, and a poly(A) tail.
Referenced in Figure 1 below, the production of mRNA drug substance is performed utilizing an in vitro chemoenzymatic process with many of the delivery issues overcome by incorporation of delivery elements3 in the drug substance such as Lipid Nanoparticles (LNP), cationic polymers and cationic polysaccharides. Today, the most widely used are LNP’s, which consist of an ionizable cationic lipid, lipid-linked polyethylene glycol, cholesterol, and a number of phospholipids, each with a specific mechanistic role.
The growth of mRNA therapeutics has inevitably led to a desire for a harmonized approach to provide quality attribute definition to RNA-based products and the analytical testing regimes necessary. Several organizations have already created such guidelines. In 2021, the WHO released an “evaluation of the quality, safety and efficacy of messenger RNA vaccines”, which provides a description of potential methods for characterization and control of various key quality attributes.4 With continued focus on mRNA vaccines, in 2023, the EMA began an initiative on the development of a guideline for five specific quality aspects of this therapeutic class which is expected to be published in 2024/2025.5
Also in 2023, the USP released a second edition of their mRNA draft guidelines which offer a relatively comprehensive inventory of methodologies for both DS and DP including analytical approaches to LNP’s.6
As with any therapeutic, the quality attributes of mRNA-based products can be segregated into categories which address purity, potency, quality, identity and safety.
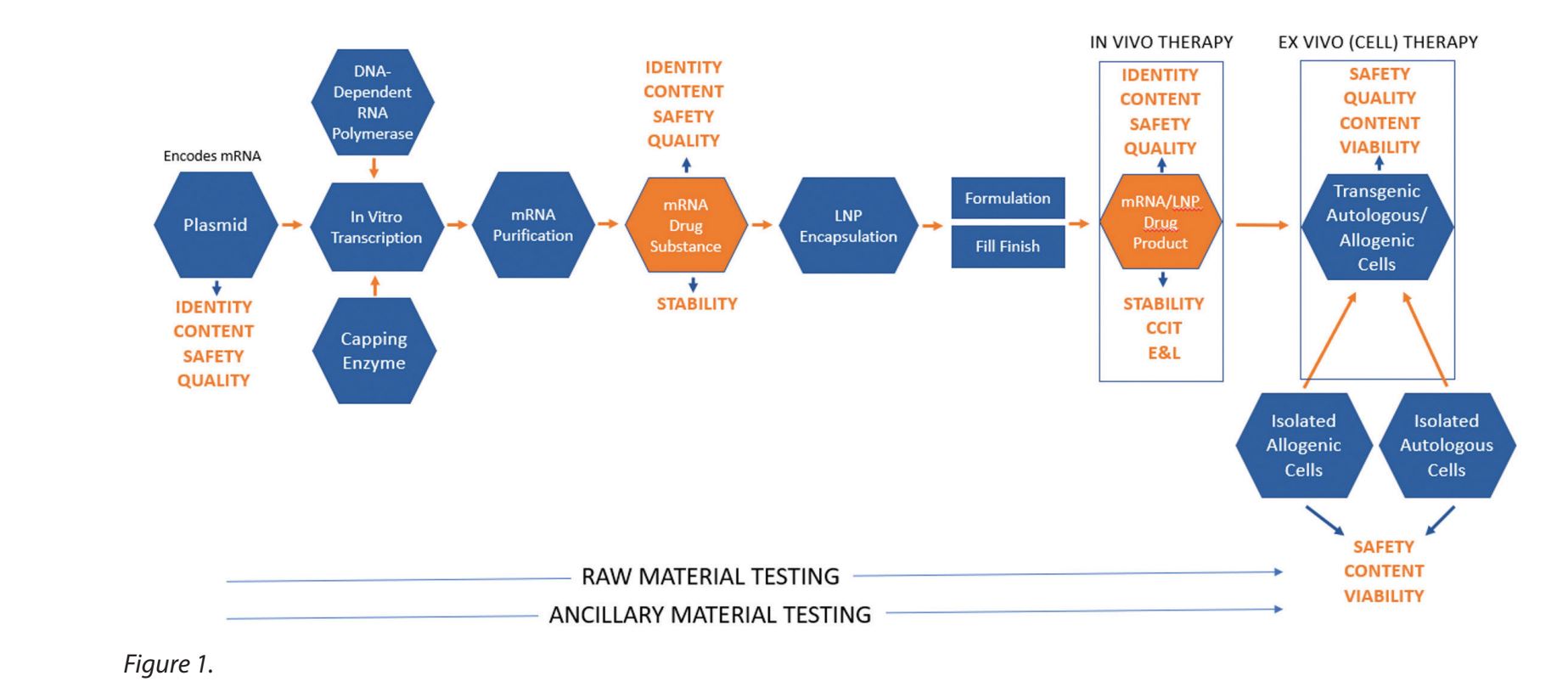
Purity: Analyses that define purity are expected to include sensitive and reliable assays for process- and product-related impurities with strict, specified upper limits. It is important that techniques are based on a wide range of physicochemical, biological and/or molecular properties. The results from forced degradation studies are often used as a guide to the choice of impurities that need to be monitored during production, release and/ or in stability protocols.
Liquid chromatographic methods are often chosen for both DP and DS. For mRNA, several modes of RP-HPLC have been described for process related impurities and assessment of 3’and 5’modifications, while SEC can provide evaluation of aggregation. Kanavarioti, 7 published an excellent review of such methods as applied to both mRNA and tRNA.
Other potential impurities such as residual DNA may be monitored and assessed by quantitative PCR while impurities such as dsRNA, which has the ability to trigger an innate immune response, may be examined by ELISA methodologies.
Potency: Potency measurements are tests of functional integrity and may be determined by in vitro and in vivo methods that provide a quantifiable biological response. For mRNA- based vaccines and other therapeutics what is measured is the expression of a functionally active antigen (encoded by the mRNA sequence). For example, using flow cytometry or immunoblotting techniques, the potency of mRNA vaccines against COVID can be determined by measurement of the encoded Spike protein (S-protein) in transfected cells.
LNP or other liposomal formulations in the finished DP can be directly tested in cell-based potency assays, as the expressed protein antigen is released and accessible in antibody- based methodologies. The observation of antigen release by itself does not guarantee that virus-neutralizing antibodies will be induced upon administration of the mRNA- based vaccine but is a valid basis for the expectation that antibodies targeting the encoded antigen will be produced.
During development, non-clinical animal models are typically used to determine if there is a dose-dependent correlation between antigen expression and antibody production. The presence of such a correlation strengthens the rationale for cell transfection- based potency assays for the lot release of nucleic acid-based vaccines. Indeed, Patel8 et al. have recently described an in vitro cell- based assay that is able to predict the potency of mRNA–LNP-based vaccines.
Quality: As with many of therapeutics, the quality of mRNA-based products is usually assessed using a variety of standard test methods, such as those described by the USP. Typically, for example, pH and appearance are recommended for the DS. The assessment of residual solvents is often part of the “quality” portfolio and can be satisfied using methodologies such as those described in USP 467 and guided by toxicity data as provided in ICH QC3(R8).
Testing may be expanded for the DP to include osmolality and extractable volume. Particle analysis is also critical, particularly for injectables and may be evaluated by a variety of techniques including Light Obscuration, Flow Imaging Microscopy, Resonant Mass Measurement and Nanoparticle Tracking Analysis.9 Raman spectroscopy can be useful for particle identification. Final product testing may also include container closure integrity and extractable and leachable assessments.
Identity: The determination of molecular identity for mRNA therapeutics can be challenging due to the structural complexity and size of these products. In some cases, modifications to structure may require the development of new techniques or at least adaptation of existing platform methods. The size and sequence of the mRNA product are critical features. Confirmation of sequence may be accomplished using methods such as Sanger sequencing, RT- PCR and other, high throughput forms of sequencing. Several methods are available for size evaluation including capillary gel (CGE), capillary electrophoresis (CE) and mass spectrometry which can be useful for tracking modified nucleotide incorporation. Such methods may require oligonucleotide “mapping”to provide the accuracy necessary for meaningful mass assignment.10
Certain specific structural features are of particular importance due to their predicted heterogeneity and effect on performance. As examples, the 5’ capping and polyA tail should be areas of analytical focus and can be monitored using well-described methods which combine sample manipulation followed by LC-MS(MS) analytics.
Similar analytical approaches to identity can be applied to the DP and the DS although the latter may require additional sample preparation. It is also important in the DP that attention is given to ID of the delivery system package, and thus, in the case of LNP’s, assessment of lipid identity, content and polydispersity as well as RNA encapsulation should all be assessed.
Safety: In the context of this article, safety testing refers to product evaluation in terms of potential microbiological contamination. For mRNA-based products using LNP delivery systems, a “conventional” biopharmaceutical testing portfolio is generally applied involving bioburden and endotoxin testing for the DS and endotoxin and sterility for the DS. All methods are described in the USP.
However, many of these testing methodologies are dated and now often challenged by regulators and the industry itself, particularly in terms of speed and sensitivity. In response, investigators such as Terayama11 have recently described an improved endotoxin method that reduces analysis times and improves accuracy. A rapid microfluidic assay has been described by Surrette12 which reduces microbiological screening to a 3hr analysis time.
As the development of mRNA therapeutics continues, the need for robust and detailed analytical examination of the products’ safety, quality, purity, potency, and identity remains critical. Strong scientific expertise and a deep understanding of the required testing techniques can help to accelerate the development process while ensuring the quality attributes of these important, life- saving treatments.
The future development and advancement of RNA-based therapies is inevitable. Machine learning is already being applied to develop predictive models and engineer de novo sequences that optimize protein expression.13 Concurrently, innovative analytical technologies will be required to support our understanding and control of these products. Recently, Gunter14 described a protocol, known as “Vax-seq” that is able to measure key mRNA quality attributes, including sequence identity, integrity, 3’-poly(A) tail length and DNA and RNA contamination in a single method.
Despite the worldwide use of vaccines based on mRNA-LNP’s, there still seems much to learn about the precise nature and structure of such products. In 2021, Brader15 reported on the location of mRNA within LNP’s revealing information about the LNP structure, nanoheterogeneity, and microenvironment.
References
- McKernan K. Helbert, Y. Kane L. McLaughlin S. Sequencing of bivalent Moderna and Pfizer mRNA vaccines reveals nanogram to microgram quantities of expression vector dsDNA per dose (2023). 10.31219/osf.io/ b9t7m.
- Wang Y-S. Kuman M. Chen G-H. et al mRNA vaccines and therapeutics: an in-depth survey of current and upcoming clinical applications. J. Biomed. Sci. 2023;30;84
- QinS. Tang X. Chen Y. et al m-RNA based therapeutics: powerful and versatile tools to combat diseases Signal Transduction and Targeted Therapy 2022;7:166
- Sheets R. Liu M. Meyer H. et al Evaluation of the quality, safety and efficacy of messenger RNA vaccines for the prevention of infectious diseases: regulatory considerations. World Health Organization 2012; WHO/ BS/2021.2402
- Concept paper on the development of a Guideline on the quality aspects of mRNA vaccines. European Medicines Agency. 2023; EMA/CHMP/ BWP/211968/2023
- Analytical procedures for mRNA vaccine quality. Available at: https://www.usp.org/sites/default/ files/usp/document/our-work/ biologics/documents/vaccine-mrna- guidelines-2.pdf.
- Kanavarioti A. HPLC methods for purity evaluation of man-made single- stranded RNAs. Sci Rep 2019;9,1019.
- Patel N. Davis Z. Hofmann C. Development and Characterization of an In Vitro Cell-Based Assay to predict Potency of mRNA-LNP-Based Vaccines Vaccines 2023; 11(7): 1224.
- Carpenter J. Fradkin A. Vessley A. Meeting biopharmaceutical analytical requirements for subvisible particle sizing and counting. European Pharm Rev 2015; Issue 5
- Vanhinsbergh C. Criscuolo A. Sutton N. Characterization and Sequence Mapping of Large RNA and mRNA Therapeutics Using Mass Spectrometry Anal. Chem. 2022;947339:7349
- Terayama M. Takahashi G. Nonoguchi M. et al. Clinical relevance of leukocyte-associated endotoxins measured by semi-automatic synthetic luminescent substrate method. Scientific Reports 2023; 13:1963
- Surrette C. B. Scherer B. 1, A. Corwin A. et al Rapid Microbiology Screening in Pharmaceutical Workflows. SLAS Technol. 2018; 23(4): 387–394
- Castillo-Hair S. Seelig G. Machine learning for designing next-generation mRNA therapeutics. Acc. Chem. Res. 2022; 55, 24−34
- Gunter H. Idrisoglu S. Swati Singh S. et al mRNA vaccine quality analysis using RNA sequencing. Nature Comm. 2023; 14, 5663.
- Brader M. Williams S. Banks J. Encapsulation state of messenger RNA inside lipid nanoparticles. Biophys. J. 2021; 120, 2766
Subscribe to our e-Newsletters
Stay up to date with the latest news, articles, and events. Plus, get special
offers from American Pharmaceutical Review delivered to your inbox!
Sign up now!