Abstract
Accumulation of lipid-laden macrophages in the arterial wall is an early characteristic of atherosclerotic lesion formation. Lipid uptake by macrophages can be studied in vitro by treating macrophages with modified (i.e. acetylated) low-density lipoprotein (LDL) and measuring the accumulated free cholesterol and cholesterol esters. Initially, a commercially available enzymatic assay for the determination of total and free cholesterol was investigated. Application of the enzymatic assay to macrophage uptake experiments proved problematic due to poor capturing of acetylated LDL (acLDL) loading (thought to be incompatible with lipid extraction methodology). In order to verify successful uptake of lipids by macrophages, an LC/MS method was developed to characterize the distribution of cholesterol and cholesteryl-esters in macrophages treated with acLDL in the absence or presence of a known Acyl-CoA:cholesterol O-acyl transferase (ACAT) inhibitor. High resolution LC/MS analyses were performed on a full scan mass spectrometer fitted with an APCI source. LC/MS was able to capture a dose-dependent increase of cholesterol ester loading that was decreased by the ACAT inhibitor DUP128. The LC/MS results guided the modification of the enzymatic assay and, after optimization, both assays yielded consistent results and allowed measurement of specific cholesteryl-esters in macrophages and their response to ACAT inhibition.
Introduction
Lipid-laden macrophages, or foam cells, are a characteristic component of atherosclerotic lesions [1]. Foam cells are typically enriched with cholesterol and ACAT-derived cholesteryl-esters. Foam cell formation can be studied in vitro by incubating macrophages with modified forms of LDL. The uptake of modified (typically acetylated or oxidized) LDL by macrophages and the subsequent intra-cellular deposition and distribution of lipids can be used to study the effects of lipid accumulation on macrophage structure and function. Uptake of acLDL by macrophages is known to result in deposition of primarily cholesterol and cholesteryl-esters (CE), particularly cholesteryl-oleate and cholesteryl-linoleate [2,3].
Measurement of cholesterol and CE has been accomplished using a variety of techniques, the most traditional of which is thin-layer chromatography (TLC). Quantitation of cholesterol and CE by TLC requires excision of bands and either quantitation by scintillation counting or by additional enzymatic means [4,5]. An alternative technique for measuring cholesterol and CE is high performance liquid chromatography (HPLC) coupled with various types of detectors. Dean and other investigators have routinely applied HPLC-UV to study the lipid content of macrophages following uptake of oxidized- or acLDL [2, 6-9]. Light scattering detection can also be used in combination with HPLC, as demonstrated by Greenspan et al., who measured cholesterol deposition in macrophages using this technique [10].
Gas-chromatography mass spectrometry (GC/MS) is an additional analytical platform that can be used for determination of various lipid classes. However, GC/MS often involves derivatization of analytes prior to analysis and is complicated by isomerization and decomposition of lipid side chains [11-13].
Because of its sensitivity and selectivity, mass spectrometry coupled to HPLC and uHPLC has become a powerful tool for determination of a broad range of polar and non-polar, small and large molecules in a variety of biological matrices. Use of LC/MS for the detection of cholesterol and CE is well-documented in the literature. Cholesterol does not respond well to electrospray ionization (ESI), but different approaches have been taken to circumvent this problem. Derivitization of cholesterol to achieve better ionization is one option. For example, Sandhoff and colleagues measured cholesterol in cells and subcellular membranes following derivitization to cholesterol-3-sulfate followed by subsequent analysis using nano-ESI/MS/MS [14]. Pentafluorophenyl derivatives of cholesterol were used to determine free cholesterol levels in atherosclerotic plaque by normal phase LC/APCI (atmospheric pressure chemical ionization)-MS/MS [15]. Both cholesterol and CE can, however, be analyzed without derivitization. Although formation of the protonated molecules is not favored using ESI, both cholesterol and CE form ammonium adducts which can be monitored for LC/MS analyses. This was demonstrated in the determination of CE by LC/ESI-MS in mouse plasma to monitor lipid assembly in vivo [16]. Liebisch et al. used ammonium adducts of CEs to characterize cell specific lipid uptake using LC/ESI-MS/MS [17]. APCI-MS also can be used for cholesterol determination. APCI-MS produces primarily an ion resulting from cholesterol water loss (m/z 369). Formation of this ion is characteristic and can reliably be used to measure cholesterol in various matrices, such as food extracts [18]. The same ion is formed upon APCI-MS analysis of CEs and is suitable for accurate determination of CEs, as demonstrated by Butovich, who characterized the CE composition of meibum using LC/APCI-MS [19]. Measurement of both cholesterol and CEs in the same analytical run is often desired but can be challenging because of the sensitivity issues associated with LC/ESI-MS and the specificity limits of LC/APCI-MS (both cholesterol and CEs produce the m/z 369 ion, therefore chromatographic separation of the two is required). One option is hydrolysis of CEs to free cholesterol followed by determination of the ratio of free cholesterol to esterified cholesterol. This approach was used by Kuo and colleagues, who used normal phase LC/APCI-MS/MS to measure free cholesterol and CEs in the same sample of atherosclerotic plaque by hydrolyzing part of the plaque sample and determining the total cholesterol content by LC/MS/MS (monitoring the pentafluorophenyl derivative of cholesterol) and comparing the results to cholesterol levels in a non-hydrolyzed plaque sample [15]. In reverse fashion, Liebisch et al. measured the ammonium adducts of CEs by LC/MS/MS in human skin fibroblast and macrophage preparations, and measured free cholesterol in parallel by first derivatizing to the cholesteryl-acetate ester [17]. In some instances, sensitivity requirements may warrant two separate analyses in order to achieve the desired results from a study. For example, measurement of cholesterol flux was accomplished by applying LC/APPI (atmospheric pressure photoionization)-MS for the determination of free cholesterol in mouse plasma, while characterizing and determining CEs in a separate analysis using LC/ESI-MS [20].
For the experiments described here, analysis of free cholesterol and CE in one analytical run was desired. Fortunately, levels of both were expected to be reasonably high in the proposed experimental system and sensitivity was not expected to be an issue. Using LC/APCI-MS (with chromatographic separation of cholesterol from CEs) we were able to accurately determine free cholesterol and CE ratios in macrophage uptake experiments. The LC/APCI-MS method was developed as an alternative and used to help optimize conditions for an enzymatic assay. The enzymatic cholesterol assay utilizes cholesterol oxidase to form hydrogen peroxide, which is then detected fluorometrically following reaction with the reagent in the presence of horseradish peroxidase. Both assays were used in parallel to characterize and optimize the lipid-loading properties of mouse peritoneal-derived macrophages. The results obtained using LC/APCI-MS closely matched those obtained using the enzymatic assay and helped to optimize and define an in vitro system for studying the lipid-uptake properties of macrophages. Ultimately, the development and implementation of an enzymatic assay was preferred over LC/MS for routine measurements due to its ease of use and fast data turn-around, both of which are critical in a drug discovery environment.
Experimental Procedures
Materials:
- Cholesteryl-oleate and cholesteryl-linoleate.
- Cholesterol-2,2,3,4,4,6-d6 and cholesteryl-2,2,3,4,4,6-d6-octadecanoate.
- HPLC grade water and methanol.
- Formic acid (98%).
- LC/MS conditions
LC/MS was performed on a benchtop LC/MS system coupled to a uHPLC pump and CTC Pal autosampler equipped with a DLW wash station. uHPLC (long method) was performed on a commercial method development column for UPLC separations heated to 65°C and operated at a flow rate of 0.6 mL/ min. Mobile phase A was 0.1% formic acid in water, mobile phase B was 0.1% formic acid in methanol. Gradient conditions were as follows: 80-100% B in 1 minute; hold 100% B for 16 minutes; re- equilibrate 0.5 minutes. Conditions for the short uHPLC method used the same mobile phase, flow and column temperature but the gradient was shortened to: 80-100% B in 0.5 minutes; hold 100% B 2.5 minutes; re-equilibrate 0.5 minutes.
Mass spectrometry was performed on a benchtop LC/MS system fitted with an APCI source and operated in positive ionization mode.Mass calibration was performed daily and MS parameters were optimized by infusing a solution of cholesteryl-oleate and adjusting parameters as needed. The ion monitored for cholesterol, cholesteryl-oleate and cholesteryl-linoleate was m/z 369.3521, representing (cholesterol – H2O +H)+. The ion monitored for the internal standards D6-cholesterol and D6-cholesteryl- octadecanoate was m/z 375.3897.
Macrophage uptake experiments and sample preparation: Thioglycollate-elicited peritoneal macrophages were harvested from male C57BL/6J mice according to Gargalovic, et al. [21]. Cells were washed in PBS containing 20% FBS and plated to standard tissue culture-treated 96 well plates at 150,000 per well in DMEM containing 20% FBS and antibiotic/antimycotic solution. After overnight incubation at 37°C/5%CO2, triplicate wells were treated in serum-free DMEM with 0, 50 or 100 ug/ml of acLDL or 100 ug/ ml acLDL containing 300 nM of the ACAT inhibitor DUP128. After overnight incubation, cells were washed thoroughly with PBS at 37°C and resuspended in 200 ul of 95%MeOH/5%CHCl3 containing 5 ug/ml D6-cholesterol (internal standard), 5 ug/ml D6-cholesteryl- ocatadecanoate (internal standard) for LCMS analysis or 200 ul MeOH for enzymatic analysis (final lipid extraction protocol for enzymatic assay). Total and free cholesterol were quantified in each sample using a commercially available enzymatic assay. Cholesterol ester concentration was calculated by subtraction of free cholesterol from total cholesterol.
Results and Discussion
Cholesteryl-oleate (CO) and cholesteryl-linoleate (CL) have been shown to constitute approximately 80% of the esterified cholesterol pool in acLDL-loaded macrophages, making them (together with free cholesterol) the focus of our studies [2-3]. When subjected to APCI-MS (+), cholesterol and CEs both produce primarily ion m/z 369, corresponding to (cholesterol-H2O+H)+ (Figure 1). Because all species form a common ion, chromatographic separation of cholesterol and the two primary CEs of interest was needed (Figure 2). Initial experiments were conducted using LC/MS with the goal of obtaining information on the accumulation of cholesterol and CEs within the macrophage, as well as how the accumulation was affected by ACAT inhibition. Results from the LC/MS assay were then used to help improve the existing enzymatic assay. A typical experiment included a negative control of untreated macrophages, macrophages treated with 100 μg/mL acLDL, and macrophages treated with 100 μg/mL acLDL in the presence of 300 nM DUP128 (an ACAT inhibitor).
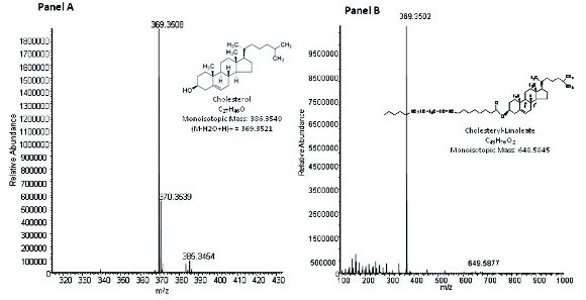
Figure 1. APCI-MS mass spectrum of cholesterol (Panel A) and cholesteryl-linoleate (Panel B). Full scan accurate mass spectra were obtained by infusion of each analyte (10 μg/mL) at 5 μL/min with mobile phase fl ow at 0.6 mL/min.
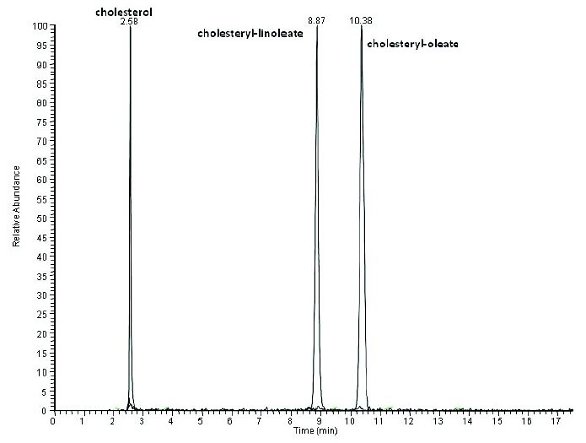
Figure 2. LC/APCI-MS chromatogram of a standard mix of cholesterol, cholesteryl-oleate and cholesteryl-linoleate (1 μg/mL each). LC/MS conditions were as described in Experimental Procedures.
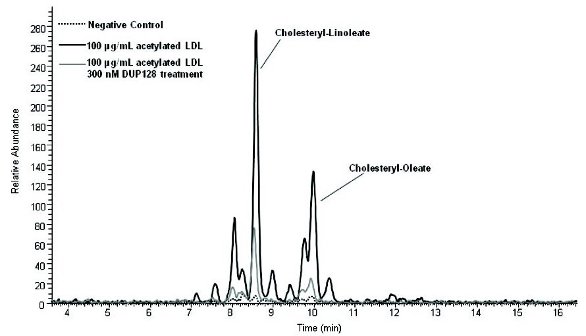
Figure 3. LC/APCI-MS chromatogram of a macrophage only (negative control) reaction, macrophage treated with 100 μg/ mL acLDL, and macrophage treated with 100 μg/mL acLDL in the presence of 300 nM DUP128. Macrophage reaction experimental details and LC/MS conditions were as described in Experimental Procedures.
Analysis of the reactions by LC/MS showed that CO and CL did appear to be the most prominent CEs formed in the acLDL-loaded-macrophages (Figure 3). As seen in Figure 3, the chromatogram is complex, with several peaks eluting around CO and CL likely representing other CEs present in the system (the cholesterol signal in the reactions is much higher than the CEs; for this reason the cholesterol peak was not included in Figure 3 in order to magnify the CE region of the chromatogram). The primary goal of these experiments was to monitor bulk changes in CE loading, therefore no further work was done to identify the unknown peaks. To verify the identity of CO and CL, a mixing experiment was performed by spiking the acLDL/macrophage sample with standards of each. Figure 4 (Panel A) shows the resulting chromatogram, and supports the peak assignments suggested. As relative changes between treatment groups were of primary interest, data were plotted as either total peak area or the peak area ratio of each component to the peak area of the internal standard. Calibration curves of each analyte were generated with standards to verify linear response (data not shown). Plotting of the peak areas of cholesterol, CO and CL demonstrated the expected profile of increased CO and CL uptake in acLDL-treated macrophages compared to the macrophage control (Figure 5). CE accumulation was partially inhibited by the ACAT inhibitor DUP128 (DUP128 had no effect on free cholesterol accumulation) as expected.
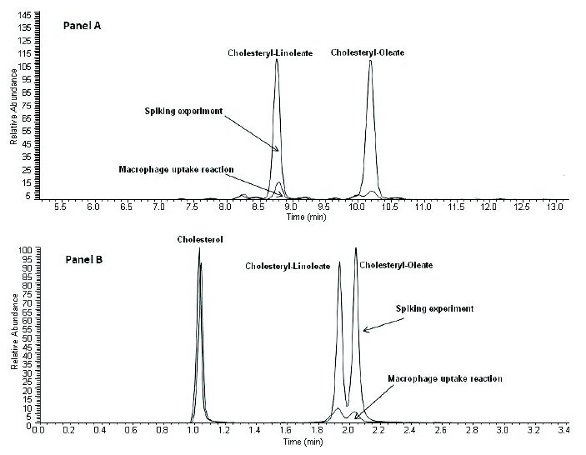
Figure 4. LC/APCI-MS chromatogram (Panel A = long LC/MS method, Panel B = short LC/MS method) of an acLDL macrophage reaction overlaid with the same reaction spiked with standards of cholesteryl-oleate and cholesteryl-linoleate. Macrophage reaction experimental details and LC/MS conditions were as described in Experimental Procedures.
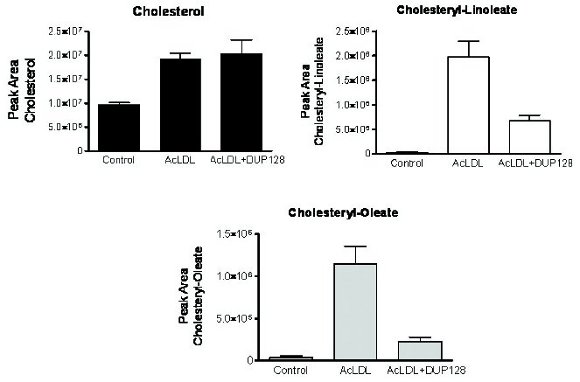
Figure 5. Summary of results obtained following LC/MS (long method) analysis of a macrophage only (negative control) reaction, macrophage treated with 100 μg/mL acLDL, and macrophage treated with 100 μg/mL acLDL in the presence of 300 nM DUP128. Macrophage reaction experimental details and LC/MS conditions were as described in Experimental Procedures. All experiments were performed in triplicate. Data is shown as the mean ± SEM.
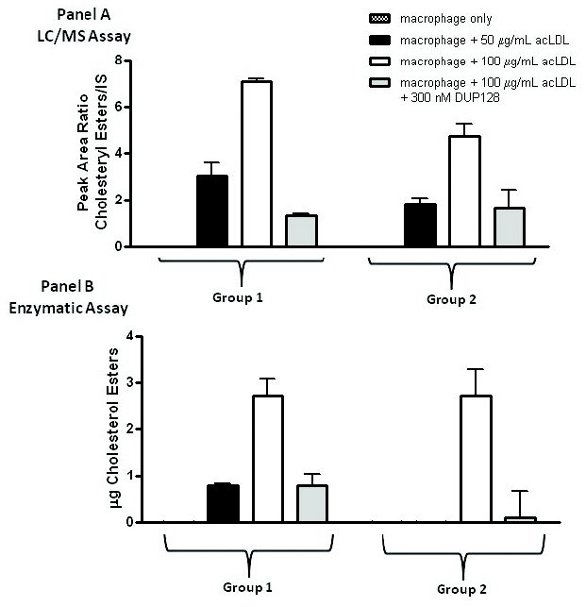
Figure 6. Panel A: Summary of results obtained following LC/MS (short method) analysis of a macrophage only (negative control) reaction, macrophage treated with 50 μg/mL acLDL, macrophage treated with 100 μg/mL acLDL and macrophage treated with 100 μg/mL acLDL in the presence of 300 nM DUP128. Macrophage reaction experimental details and LC/MS conditions were as described in Experimental Procedures. Panel B: summary of results obtained following enzymatic assay analysis of samples described in Panel A. Macrophage reaction experimental details and enzymatic assay conditions were as described in Experimental Procedures. All experiments were performed in triplicate. All data were normalized and are shown as the mean lipid value per mg of cellular protein ± SEM.
The decision was made to shorten the LC method, with the goal of maintaining separation between cholesterol and the CEs, but not necessarily between CO and CL. The logic was that a total sum of the peak areas taken from the major CEs present in the reactions would accurately represent the major CE population in the system. Figure 4 (Panel B) shows a repeat of the mixing experiment illustrated in Panel A analyzed using a shorter (3.5 minute) method. Combining the total peak area of CO and CL led to similar results as was obtained by integrating each peak separately. Figure 6 shows the results from an experiment that included untreated macrophages, macrophage treated with acLDL at 50 and 100 μg/ml, and macrophages treated with acLDL (100 μg/ml) in the presence of 300 nM DUP128. This particular experiment consisted of two animal groups from which peritoneal macrophages were harvested, designated here as Group 1 and Group 2. Panel A is a summary of the results using the LC/MS method, where the peak area of the major CE region of the chromatogram was combined. Using this method, the accumulation of CEs was seen to increase in parallel with increasing concentrations of acLDL, and the accumulation was partially inhibited by DUP128. The LC/MS results agreed closely with results obtained from an identical experiment analyzed in parallel using an improved enzymatic assay (Figure 6, Panel B). Both methods have shown to be highly reproducible in similar follow- up experiments.
Conclusions
Characterization of the lipid uptake properties of macrophages is an important component of understanding the series of events that lead to accumulation of plaque and eventual complications associated with atherosclerotic disease. We used LC/APCI-MS in parallel with a commercially available enzyme-based cholesterol assay to characterize and validate an acLDL treated macrophage system. The results obtained using LC/APCI-MS matched those obtained using the enzymatic assay, and verified that both methods are suitable for monitoring the CE uptake in macrophages treated with acLDL.
Acknowledgements
The authors thank Ms. Debra Search for isolation and preparation of mouse macrophages.
References
- Stary HC, Chandler AB, Glagov S, Guyton JR, Insull W. Jr., Rosenfeld ME, Schaffer SA, Schwartz CJ, Wagner WD and Wissler RW (1994) Circulation 89:2462-2478.
- Brown AJ, Mander EL, Gelissen IC, Kritharides L, Dean RT and Jessup W (2000) J Lipid Research 41:226-236
- Cignarella, A et al, Arteriosclerosis, Thrombosis and Vasc Biol (1998) 18:1322
- Maor I and Aviram M (1994) J Lipid Research 35:803-819
- Jialal I and Chait A (1989) J Lipid Research 30:1561-1568
- Kritharides L, Jessup W, Gifford J and Dean RT (1993) Anal Biochem 213(1):79-89.
- Kritharides L, Jessup W, Mander EL and Dean RT (1995) Arterio Throm Vascular Biology 15:276-289.
- Greenspan MD, Lo C-Y Lee, Hanf DP and Yudkovitz JB (1988) J Lipid Research 29:971-976.
- Vercaemst R, Union A and Rosseneu M (1989) J Chromatography 494:43-52.
- Greenspan P, Yu H, Mao F and Gutman RL (1997) J Lipid Research 38:101-109
- Morris LJ, Holman RT and Fontell K (1960) J Lipid Research 1:412-420.
- Mjøs S, Meier S and Grahl-Nielsen O (2006) Eur J Lipid Technol 108:315-322.
- Fournier V, Destaillats F, Juaneda P, Dionisi F, Lambelet P, Sebedio J-L et.al (2006) Eur J Lipid Technol 108:33-42.
- Sandhoff R, Brügger B, Jeckel D, Lehmann WD and Wieland FT (1999) J Lipid Research 40:126-132.
- Kuo M-S, Kalbfleisch JM, Rutherford P, Gifford-Moore D, Huang X-D, Christie R, Hui K, Gould K and Rekhter M (2008) J Lipid Research 49:1353-1363.
- McLaren, DG, He T, Wang S-P, Vendoza V, Rosa R, Gagen K, Bhat G, Herath K, Miller PL, Stribling S, Taggart A, Imriglio J, Liu J, Chen D, Pinto S, Balkovec JM, DeVita RJ, Marsh DJ, Castro-Perez JM, Strack A, Johns DG, Previs SF, Hubbard BK and Roddy TP (2011) J Lipid Research 52:1150-1161.
- Liebisch G, Binder M, Schifferer R, Langmann T, Schulz B and Schmitz G (2006) Biochimica et Biophysica Acta 1761:121-128.
- Raith K, Brenner C, Farwanah H, Müller G, Eder K and Neubert RHH (2005) J Chromatography A 1067:207-211.
- Butovich IA (2010) Steroids 75:726-733.
- Castro-Perez J, Previs SF, McLaren DG, Shah V, Herath K, Bhat G, Johns DG, Wang SP, Mitnaul L, Jensen K, Vreeken R, Hankemeier T, Roddy TP and Hubbard BK (2011) J Lipid Research 52:159-169.
- Gargalovic P and Dory L (2001) J Biol Chem 276(28):26164-26170
Author Biographies
Joelle M. Onorato received a Ph.D. in Biochemistry from the University of South Carolina, followed by post-doctoral work at Cornell University. She is currently a Senior Research Investigator in Mass Spectrometry/Bioanalytical and Discovery Analytical Sciences within Research & Development at Bristol-Myers Squibb Company.
Sam Hellings received an AB in Biology from Princeton University and an MS in Marine Biology and Biochemistry from the University of Delaware. He is currently a Senior Research Scientist in Cardiovascular Discovery within Research & Development at Bristol-Myers Squibb Company.
Peter S. Gargalovic. received his Ph.D. in Biochemistry and Molecular Biology from the University of North Texas Health Science Center at Fort Worth, followed by post-doctoral fellowship at UCLA. He is currently a Senior Research Investigator in Cardiovascular Drug Discovery within Research & Development at Bristol-Myers Squibb Company.
Robert Langish received a BS in Chemistry from St. Joseph’s University and an MS in Analytical Chemistry from Drexel University. He is currently a Research Scientist in Mass Spectrometry/Bioanalytical and Discovery Analytical Sciences within Research & Development at Bristol-Myers Squibb Company.
Petia Shipkova received a Ph.D. in Chemistry from the University of Florida, followed by post-doctoral work at Cornell University She is currently a Principal Scientist in Mass Spectrometry/Bioanalytical and Discovery Analytical Sciences within Research & Development at Bristol-Myers Squibb Company.