Pauline van der Wijst – Janssen, Product Application Specialist in DFE Pharma’s Inhalation division; Ross Blezard,Product Application Specialist in DFE Pharma’s Biopharma division
The administration route of a drug is a critical decision during medicine development: it can decide the success or failure of the drug product. During the development process clinical factors (such as efficacy in humans and patient compliance) as well as commercial factors (such as development and manufacturing costs) have to be taken into account. There are many defined routes of administration, which can be loosely classified into three groups: Enteral, where a medicine is delivered through the gastrointestinal tract, for example with tablets and capsules. Parenteral, which in strict terms would indicate any route other than the enteral route, however, is commonly associated with injectable routes such as intravenous (IV), intramuscular (IM), intradermal (ID) or subcutaneous (SC). Whilst parenteral administration routes are effective from a clinical standpoint, they are not considered patient-friendly administration routes (specifically IV). The final administration route we can term as “other”; this is where inhalation and pulmonary delivery may offer a patient-friendly alternative to IV.
Pulmonary delivery of a water-soluble API has a multitude of benefits over other established patient-friendly routes of administration, like oral delivery. The large surface area available for absorption in the lungs typically results in a rapid and predictable onset of action. Pulmonary delivery largely avoids the first pass metabolism, meaning that higher concentrations of the active drug are available at the site of action. Additionally, drug delivery to the targeted tissue area can result in fewer side effects.
Pulmonary administration is currently an essential route in the treatment of respiratory conditions. Dry powder inhalers (DPIs), are non-invasive devices designed to deliver dry powder to the lungs upon inhalation. DPIs have been used in the treatment of diseases, such as asthma and chronic obstructive pulmonary disease (COPD), for decades. Pulmonary drug delivery is a growing area with new products designed to treat everything from diabetes to neurological conditions currently making their way through the development pipeline and into clinical practice.
DPIs have advantages over other forms of pulmonary administration devices like nebulizers, metered dose inhalers and soft mist inhalers (see Table 1). They are, for example, portable, easy to use, and contain the formulation in a dry form. What’s more, they have a relatively low carbon footprint because, unlike pressurized metered-dose inhalers (pMDI), they do not contain a propellant.
Trends in the Industry
In order to expand and fully utilize the inhaled route there is a requirement to deliver high drug doses (for example in the case of antibiotics). Additionally, with the increasing popularity of biologics and complexity of dose adjustment, pulmonary delivery can be an ideal platform to accommodate the formulation of this class of complex molecules.
Delivering High Doses
The efficacy of an inhaled drug heavily relies on the deposition of the active pharmaceutical ingredient (API) into the lungs, and it is the aerodynamic particle size distribution (APSD) that defines how powders behave in an airstream during inhalation.
Generally, API particles after crystallization require size reduction to allow inhalation delivery. The optimal aerodynamic particle size to reach the bronchial region is <5 μm, with the majority of the particles being 2-3 μm.2Traditionally, top-down processes, like micronization, are used for the particle size reduction. This approach presents flowability and dispersibility challenges. Particles with lower particle size have high surface area and hence high adhesive forces. Overcoming these challenges relies on the careful design of both the powder blend and the DPI device.
The most common approach to address these challenges is to formulate APIs with a carrier excipient, usually lactose monohydrate. The carrier has coarser particles than the API, typically between 50 to 100 μm in diameter, and form a loose bond with the drug.2 As the formulation is inhaled, the drug detaches from the carrier particle, allowing the drug to be delivered to lungs and the carrier to be deposited in the throat and swallowed. Using a carrier also aids fluidization and dispersion, improving the flow of the formulation to aid processing steps such as device filling and dose metering.
This approach is referred to as “carrier-based” formulation and is well established for the majority of formulations and it works very well. Both the powder blend and the DPI device must be carefully designed to ensure detachment of the micronized drug from the carrier excipient on inhalation.
DPIs are typically designed to deliver API doses of microgram quantities. For example, the Ellipta® product range contains 40 µg of vilanterol trifenatate and 74.2 µg umeclidinium bromide with a total powder mass of 12.5 mg (mainly lactose monohydrate).3 For some APIs however, higher doses are required to achieve the desired therapeutic effect. As drug loading increases, it becomes increasingly challenging to maintain the correct adhesion-cohesion balance, whilst avoiding powder blend challenges such as uniformity and segregation. In the traditional carrier-based model, an increased delivered dose therefore requires a corresponding increase in excipient, which may come with some challenges. Large volumes of powder threaten effective drug-carrier separation, and can be difficult to inhale, threatening dose uniformity. Large powder volumes are also associated with side effects, the most commonly reported being coughing. Aside from being unpleasant, in people with pulmonary diseases, such a lung cancer or Cystic Fibrosis (CF), coughing can irritate and damage the lung, adding to the likelihood of adverse outcomes. In addition, introducing large volumes of powder to the lungs increases the chances of the drug being removed via mucociliary clearance.4
The Rise of Biologics
Biologics are a class of medications that offer unique treatment options for many of the world’s most challenging diseases. The terms biologics and biopharmaceuticals cover a broad class of molecules that include, among others, peptides, proteins, nucleic acids as well as blood, tissue, cell and gene products. Biologics have the potential to change patient lives significantly. For example, in some types of cancer, biologics constitute the first new treatments in decades. In fact, biologics appear to show promise across almost all disease areas as well as many diagnostic applications. The expectation is that biologics will significantly overtake innovative small molecules sales over the next five years. By 2027, biologics sales are forecasted to exceed small molecules sales by $120 billion.5
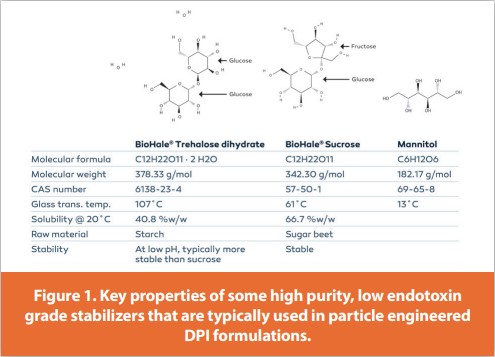
Biologics are a complex class of drug. They typically have a poor stability profile (compared to small molecules), and are often sensitive to temperature, light and pH changes. Additionally, they have poor permeability through the intestinal epithelium and are susceptible to enzymatic degradation in the gastrointestinal tract.6 This means that, historically, biologics have been administered parenterally, despite this being invasive and inconvenient for the patient, and resource hungry for healthcare systems.7
In recent years, inhaled formulations and DPI systems have become widely accepted as an alternative to the SC and enteral (oral) administration of therapeutic peptides and proteins. They avoid the potential of poor absorption and high metabolism in the gastrointestinal tract, and the first-pass effects in the liver. In addition, inhalation therapy, unlike injection delivery, is pain free making it more convenient for patients. Whilst this is a highly active area of research in general terms, for smaller biologics, such as insulin, systemic delivery is possible and for larger molecules, such as monoclonal antibodies (mAbs), local treatment of the lung tissue is possible.
Traditional ‘carrier-based’ DPI drug delivery, however, is not always suited to the delivery of these promising molecules, particularly those that require additional stabilization. Stresses from size reduction, but also during storage and handling, can reduce the activity of biologics. Alternative processing strategies are required, and the use of excipients to stabilize the biologic and improve powder properties may be required.
Excipients in Spray Drying
Particle engineering offers an alternative route, which is especially suitable for high-dose and biological formulations. Spray drying is the most commonly used technology for particle engineering in DPI, although also other alternatives exist, like spheroid formation or freeze drying. Spray drying can be used to modify the particle morphology of the API, resulting in a reduced aerodynamic diameter without having poor flow and poor aerosolization properties. The physical properties of spray-dried particles are highly dependent on the process parameters used and the composition of the spraying matrix. Without excipients, material properties are highly dependent on the properties of the drug. Unfortunately, very few molecules can be directly spray-dried with the desired physical properties without the addition of excipients. Excipients are added to improve the general powder handling, or they can be added to protect the API from external stresses.
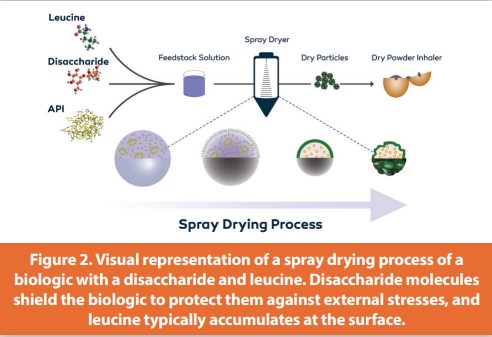
Whilst many excipients are described for this application in literature, three excipients in particular show great promise for use as a filler in the spray-drying processes. These are trehalose and sucrose, which are non-reducing crystalline disaccharides, and the sugar alcohol mannitol. All three provide improved compatibility with biomolecules compared to reducing sugars. They are not easily hydrolyzed by acid, and they can help these complex molecules maintain their native conformation. In addition, they can enable modification of the resulting powder’s physical properties. They also have an excellent safety profile and are commonly used as lyo- and cryoprotectants in parenteral administration of therapeutic proteins.
Trehalose and sucrose are particularly attractive due to their high glass transition temperature. This enables the formation of a glassy amorphous matrix, which in turn may be particularly suited to maintaining the stability and activity of biomolecules. In addition, when compared to other disaccharides, trehalose is highly stable under low-pH conditions.
Because trehalose can function as a particle-matrix/stabilizing agent, it is a promising excipient for the delivery of biomolecules, such as peptides and proteins. Sucrose could also play a role in this area, and it has a demonstrated utility as an excipient for peptide and protein delivery, for example it is already being utilized in many biologic formulations, including COVID vaccines.
Mannitol has a much lower glass transition temperature, when compared to sucrose and trehalose, typically reported to be approximately 13°C. The impact of this is that it tends to crystalize after spray drying. The transition from an amorphous material to crystalline material may introduce stresses that can damage sensitive molecules, such as biologics. However, many molecules can tolerate these stresses, and in some cases, it may be preferable to have the more thermodynamically stable crystalline form. If an amorphous glass is required, then the crystallization of a matrix containing mannitol can be impeded with the addition of further excipients such as glycine and inorganic salts. Additionally, mannitol has a proven application in pulmonary delivery due to its use in Exubera®, the first inhaled insulin dry powder inhaler that was available in the market between 2006 and 2008.8
It should be noted that the three mentioned excipients would typically not be used in isolation; amino acids, and specifically leucine types, are commonly utilized in academic research to enhance the aerosolization properties of spray dried particles for pulmonary drug delivery. During the spray drying process, leucine molecules accumulate at the surface of droplets with their hydrophobic part facing the gas phase. When the concentration of leucine increases at the surface and supersaturation is achieved, leucine crystallizes at the outer surface, forming a shell. This benefits the aerosolization performance, as it results in lower cohesion forces within the particles. Figure 2 shows a graphical representation of the spray drying process of a biologic with a disaccharide and leucine. Disaccharide molecules shield the biologic, while leucine accumulates at the surface and has more impact on the physical surface properties of the spray-dried powder.
Embracing the Potential of Next-Generation Inhaled Medications
Drug developers are expanding the use of inhaled medications in a bid to tackle unmet medical needs. However, with high-dose medications and biologics being unsuited to the carrier-based DPI model, this new dawn requires new formulation processing techniques.
Spray drying is emerging as one of the most promising carrier-free technologies and has already yielded important results. Careful consideration of excipient use within this emerging area of interest can help developers create bespoke formulations that meet the characteristics of the API and the needs of the patient alike.
As discussed in this article, the field of pulmonary delivery is expanding from small molecule delivery to biologics and from low dose to high dose formulations. The authors believe that this is just the start in targeting the delivery of new and existing medicine to the lungs. This shift is occurring now, for example, inhaled cannabinoids can deliver higher efficacy at lower doses than orally delivered products. This approach has shown some promise in therapy areas, such as cancer treatment-associated nausea, depression, anxiety, migraine, and pain, among others.9 Researchers in lung cancer,10 pulmonary hypertension,11 and Alzheimer’s disease12 are also investigating the benefits of pulmonary delivery. All in all, it’s an exciting and promising moment in this field.
References
- Jardim, J. R., & Nascimento, O. A. (2019). The importance of inhaler adherence to prevent COPD exacerbations. Medical Sciences, 7(4), 54. https://www.mdpi.com/2076- 3271/7/4/54
- Peng, T., Lin, S., Niu, B., Wang, X., Huang, Y., Zhang, X., ... & Wu, C. (2016). Influence of physical properties of carrier on the performance of dry powder inhalers. Acta pharmaceutica sinica B, 6(4), 308-318. https://www.ncbi.nlm.nih.gov/pmc/articles/ PMC4951591/
- Shur J., Price R., Lewis D., Young P. M., Woollam G., Singh D., Edge S., From single excipients to dual excipient platforms in dry powder inhaler products, International Journal of Pharmaceutics, Volume 514, Issue 2,2016, https://www.sciencedirect.com/science/ article/pii/S037851731630446X
- Air Physio. How Does Mucociliary Clearance Work – Mucus Clearance and Removal. (2018). Available at: https://www.airphysio.com/mucociliary-clearance-and-removal/
- GlobalData, Future of Pharma – Looking Ahead to 2022: https://store.globaldata.com/ report/future-of-pharma-insight-analysis/
- Liang, W., Pan, H. W., Vllasaliu, D., & Lam, J. K. (2020). Pulmonary delivery of biological drugs. Pharmaceutics, 12(11), 1025. https://www.mdpi.com/1999-4923/12/11/1025
- Thomas, F. (2021). Taking a Breath: Advances in Inhaled Biologics The inhaled route of administration for biologics is experiencing renewed interest, particularly in light of the COVID-19 pandemic. BIOPHARM INTERNATIONAL, 34(4), 18-+.https://www. biopharminternational.com/view/taking-a-breath-advances-in-inhaled-biologics
- Healy A. M., Amaro M. I., Paluch K. J., Tajber L., Dry powders for oral inhalation free of lactose carrier particles, Advanced Drug Delivery Reviews, Volume 75, 2014, https://www. sciencedirect.com/science/article/pii/S0169409X14000702
- National Academies of Sciences, Engineering, and Medicine. (2017). Therapeutic effects of cannabis and cannabinoids. In The health effects of cannabis and cannabinoids: The current state of evidence and recommendations for research. National Academies Press (US). https://www.ncbi.nlm.nih.gov/books/NBK425767/
- Rosière, R., Berghmans, T., De Vuyst, P., Amighi, K., & Wauthoz, N. (2019). The position of inhaled chemotherapy in the care of patients with lung tumors: clinical feasibility and indications according to recent pharmaceutical progresses. Cancers, 11(3), 329. https:// www.ncbi.nlm.nih.gov/pmc/articles/PMC6468657/#:~:text=associated%20with%20 chemotherapy.-,Inhaled%20chemotherapy%20has%20been%20proved%20to%20be%20 feasible%20and%20safe,systemic%20toxicities%20are%20consequently%20reduced.
- Hill, N. S., Preston, I. R., & Roberts, K. E. (2015). Inhaled therapies for pulmonary hypertension. Respiratory Care, 60(6), 794-805. https://pubmed.ncbi.nlm.nih.gov/26 070575/#:~:text=Accordingly%2C%20a%20number%20of%20inhaled,as%20a%20 continuously%20nebulized%20medication.
- Alzheimer’s Society. New research shows promise for Phase 2/3 trial of inhaled insulin in people with MCI or mid Alzheimer’s disease – Alzheimer’s Society comment. (2019). Available at:https://www.alzheimers.org.uk/news/2019-07-17/new-research-shows[1]promise-phase-23-trial-inhaled-insulin-people-mci-or-mid
Author Biographies
Pauline van der Wijst-Janssen is a Product Application Specialist at DFE Pharma. She has been working on application development of excipients based upon fundamental knowledge of excipients and powder physics. She joined DFE Pharma in 2017 and worked as a product developer and application specialist on multiple OSD and DPI projects. Pauline holds a Master’s Degree (cum laude) in Physical Chemistry from the Radboud University in Nijmegen, with an additional specialization in Science, Management and Innovation. Since April 2022, she is also affiliated with the University of Groningen with a PhD student position.
Ross Blezard is a Product Application Specialist for Biopharmaceuticals. He brings extensive experience in the development of inhalation drug products and material characterization, and has previously held positions including Head of formulation development for a UK based CDMO and Senior Scientist (Physical sciences) for a global leader in the food and pharmaceutical industries.
Subscribe to our e-Newsletters
Stay up to date with the latest news, articles, and events. Plus, get special
offers from American Pharmaceutical Review delivered to your inbox!
Sign up now!