Introduction
The establishment of consistent safety and efficacy of parenteral pharmaceutical products is a key goal in GMP operations. Within the spectrum of activities that support GMP operations, the control of bioactive contaminants is a critical concern. Practically, and perhaps principally, this has meant sterility and within-specification endotoxin burdens. Over the last seven decades, tests for bacterial endotoxin burdens have developed from exclusively animal-based bio-assays to LAL (Limulus Amebocyte Lysate), or LAL-derivative, biochemical assays and mammalian cell-based assays. These methods have been enshrined in national and international regulatory requirements and have performed extremely well in maintaining a high level of endotoxin-related safety in modern parenterals. This success has not engendered complacency, however, and pharmaceutical science relating to bioactive contaminants has moved forward to identify other bioactive materials whose uncontrolled presence in parenterals can potentiate risk to safety and efficacy. One such commonly encountered bioactive is (1→3)-β-D-Glucan (BG).1
(1→3)-β-D-Glucan: Structure
(1→3)-β-D-Glucan is a polymer of glucose that is produced by almost all fungi, where it can comprise up to 50% of the cell wall dry weight, and by some bacteria and algae.2 It is also a minor component of plant tissue (callose). BG is diverse in molecular weight, water solubility, branching, and biological activity.2 An image of fungal BG is presented in Figure 1. It consists of a (1→3)-β-linked polyglucose backbone with a (1→6)-β-linked branch point leading to another (1→3)-β-linked polyglucose chain. More complicated structures may include a variety of branch linkages including (1→2)-β- and (1→4)-β- linkages as well as other substituents. Bioactivity has been linked to many diverse BG structures.3,4 In particular, the least water soluble particulate forms are associated with more potent immunological activity.
Figure 1.(1→3)-β-D-Glucan: Bioactivity
BG is considered a pathogen-associated molecular pattern (PAMP) and as such is a signal of infection to the innate immune system. Its biological activity is exerted through binding to BG receptorbearing cells of the innate immune system, as well as to epithelial cells of certain organs (see Table 1). It binds to a number of receptors including Dectin-1, Complement Receptor-3, Lactosylceramide, and scavenger receptors.5 Such binding elicits the activation of signal transduction cascades, leading to diverse immune effects such as the production of pro-inflammatory cytokines, occasional down-regulation of inflammatory response, synergistic increase of inflammatory response, catastrophic, fatal immunological “storms,” and stimulated cytolytic attack.6,7 BG immunoreactivity is the subject of a rich and growing library of literature which illustrates the potential of uncontrolled BG contamination in parenterals to influence biological responses.
Table 1. Examples of cell types with (1→3)-β-D-Glucan receptors
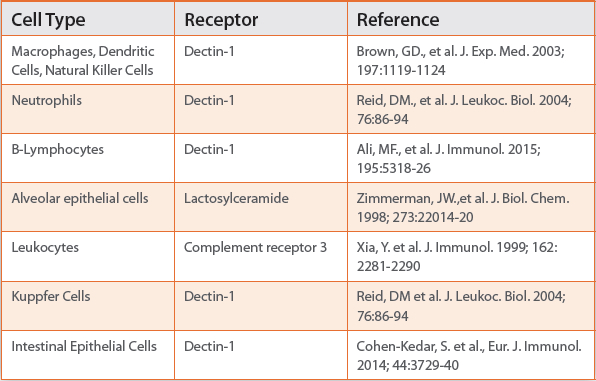
Human exposure to BG occurs, principally, in three ways: Invasive fungal disease (IFD), iatrogenic contamination, and translocation from the intestinal tract. Normal levels of circulating BG, as measured with the Fungitell IVD kit (Associates of Cape Cod, Inc.) have been demonstrated to be < 60 pg/mL.8 Levels ≥ 80 pg/mL, in at-risk patients, are considered supporting evidence of invasive fungal disease.9 Fungal BG have been demonstrated to trigger a variety of immune responses in the setting of infection, provoking activation of immune effector cells, elicitation of pro-inflammatory cytokines, enhancing antibody-dependent anti-tumor cytolytic response, and, in the presence of Toll-like Receptor ligands including endotoxin, the promotion of synergistically elevated, pro-inflammatory responses.9,10 As a result of the observed immune stimulus, BG administration has been, and continues to be, evaluated as a therapeutic in a variety of health care applications, including as an anti-cancer drug.12 Certain BG formulations are licensed anti-cancer drugs in Japan and China.
(1→3)-β-D-Glucan: Sources of Contamination
Modern pharmaceutical processes include a number of upstream and downstream unit operations that entail risk of BG contamination. These include fermentation (growth media, fungal or yeast-based recombinant cells), coarse filtration (cellulosic filtration media), processing aids (high concentration sucrose or other saccharides), diafiltration and concentration (concentration of high molecular weight BG contaminants), formulation excipients (substituted cellulose, saccharides), and sterile filtration (cellulosic filters).13-16
(1→3)-β-D-Glucan: A Confounder of LAL-based Endotoxin Detection
The most commonly utilized methods of endotoxin measurement are LAL-based. However, the LAL cascade is bi-specific, reacting to both lipopolysaccharide and BG (Fig. 2). For BG, it is the (1→3)-β-linked sequences that allow detection utilizing LAL-based reagents. The combined interactions of LPS and BG with the LAL cascade are complex and the presence of BG has been demonstrated to have a synergistic effect upon LAL activation.17
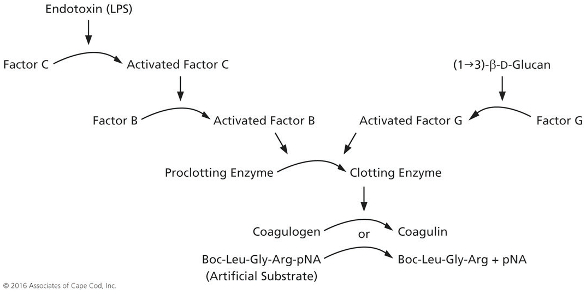
Figure 2.
Accordingly, endotoxin measurements made on materials that are contaminated with BG run the risk of over-estimating the actual endotoxin present in the test material. In order to eliminate positive interference from contaminating BG, LAL reagent manufacturers typically offer specific BG-activation blocking buffers or reagents insensitive to BG. While this approach has the effect of masking the potential presence of bioactive BG, pharmaceutical manufacturers may miss information that could be important to the understanding of product performance and adverse events in clinical use.
(1→3)-β-D-Glucan: Strategies for Contamination Prevention and BG Removal
The control of BG contamination in parenterals may be reduced to two approaches. The first involves the screening of raw materials and process unit operations’ equipment to identify and mitigate BG introduction. The second is the utilization and validation of process-based BG removal. The first approach involves characterization of raw materials, equipment leachates, and excipients for BG content. This is accomplished by using commercially available BG-specific reagents to quantitate BG burdens. These are, typically, LAL-based reagents whose preparation has inactivated or removed their capacity to become activated by lipopolysaccharide. Processrelated raw materials and unit operations characterization with these reagents permits the addition of BG specifications to materials and to process equipment selection and preparation. An example of this approach is presented by Anderson et al, 2002.15
Fermentation products which use fungal organisms will result in the broth becoming infused with BG from the fungal cell walls. In addition, media components such as sugars, yeast extract, and plant origin components will often contain significant levels of BG. The use of crude yeast-derived media components in mammalian cell culture will introduce BG at the beginning of the production process.12 Depth filtration used early in recovery processes also represents a BG contamination risk.18 Cellulose-containing depth filters leach large quantities of BG, a plant tissue component.19 As a result, many recombinant biologics and blood fractionation products develop significant BG burdens which vary from lot to lot.15,20 Extensive rinsing prior to use can reduce the levels leached during filtration operations. High concentration sugars, used as a processing aid in protein purification can also contribute unwanted BG. This material may be retained and concentrated in downstream diafiltration and concentration operations.21
Summary
BG is nearly ubiquitous in nature and may be present in the raw materials and process equipment used in the manufacture of parenteral drugs. The uncontrolled presence of bioactive BG in parenteral pharmaceuticals represents a potential source of endotoxin specification excursions, variable product performance, and adverse events, especially with immunologically active biologicals. The availability of BG-specific, LAL-based reagent kits provides facile methods to measure BG burdens. Biologics manufacturers are now beginning to identify sources of BG burdens in their manufacturing processes and are characterizing and validating its removal. At a minimum, BG burdens should be characterized, controlled, and established safe levels should not be exceeded.
References
- Novak M, Vetvicka V. Glucans as biological response modifiers. Endocr. Metab. Immune. Disord. Drug Targets. 2009;9:67-75.
- Finkelman, M., Tamura, H. 2005 Detection and measurement of (1→3)-β-Dglucan with Limulus amebocyte lysate-based reagents. In, “Toxicology of (1→3)-β-D-glucan: Glucans as a marker for fungal exposure.” Ed. Young, Shih- Houng and Castranova, Vincent. Taylor and Francis Group, Boca Raton.
- Adams EL, Rice PJ, Graves B, Ensley HE, Yu H, Brown GD, Gordon S, Monteiro MA, Papp-Szabo E, Lowman DW, Power TD, Wempe MF, Williams DL. Differential high-affinity interaction of dectin-1 with natural or synthetic glucans is dependent upon primary structure and is influenced by polymer chain length and side-chain branching. J. Pharmacol. Exp. Ther. 2008;325:115-23.
- Okazaki M, Adachi Y, Ohno N, Yadomae T.., Structure-activity relationship of (1→3)-β-D-glucans in the induction of cytokine production from macrophages, in vitro. Biol. Pharm. Bull. 1995;18:1320-1327.
- Molecular Legentil L, Paris F, Ballet C, Trouvelot S, Daire X, Vetvicka V, Ferrières V. Interactions of β-(1→3)-Glucans with Their Receptors. Molecules. 2015;20:9745-66.
- Novak M, Vetvicka V. Beta-glucans, history, and the present: immunomodulatory aspects and mechanisms of action. J Immunotoxicol. 2008;5:47-57.
- Vannucci L, Krizan J, Sima P, Stakheev D, Caja F, Rajsiglova L, Horak V, Saieh M. Immunostimulatory properties and antitumor activities of glucans (Review). Int. J. Oncol. 2013;43:357-64.
- Odabasi Z, Mattiuzzi G, Estey E, Kantarjian H, Saeki F, Ridge RJ, Ketchum PA, Finkelman MA, Rex JH, Ostrosky-Zeichner L. Beta-D-glucan as a diagnostic adjunct for invasive fungal infections: validation, cutoff development, and performance in patients with acute myelogenous leukemia and myelodysplastic syndrome. Clin. Infect. Dis. 2004;39:199-205.
- Karageorgopoulos DE, Vouloumanou EK, Ntziora F, Michalopoulos A, Rafailidis PI, Falagas ME.
- β-D-glucan assay for the diagnosis of invasive fungal infections: a metaanalysis. Clin. Infect. Dis. 2011;52:750-70. Vetvicka V. Glucan-immunostimulant, adjuvant, potential drug. World J. Clin. Oncol. 2011;2:115-9..
- Kikkert R, Bulder I, de Groot ER, Aarden LA, Finkelman MA. Potentiation of Tolllike receptor-induced cytokine production by (1→3)-β-D-glucans: implications for the monocyte activation test. J. Endotoxin Res. 2007;13:140-9.
- Vetvicka, V and Vetvickova, Glucan Supplementation Has Strong Anti -melanoma Effects: Role of NK Cells. J. Anticancer Res. 2015; 35:5287-5292
- Gefroh E, Hewig A, Vedantham G, McClure M, Krivosheyeva A, Lajmi A, Lu Y. Multipronged approach to managing beta-glucan contaminants in the downstream process: control of raw materials and filtration with chargemodified nylon 66 membrane filters. Biotechnol. Prog. 2013;29:672-80.
- Jiang C, Scherfner S, Dick LW Jr, Mahon D, Qiu D, Cheng KC, Shukla AA. Demonstrating β-glucan and yeast peptide clearance in biopharmaceutical downstream processes. Biotechnol. Prog. 2011;:442-50
- Buchacher, A., et al., Elevated Endotoxin Levels in Human Intravenous Immunoglobulin Concentrates Caused by (1→3)-β-D-glucans. PDA J. Pharm. Sci. Tech. 2010;64:536-544
- Anderson J, Eller M, Finkelman M, Birx D, Schlesinger-Frankel S, Marovich M. False positive endotoxin results in a DC product caused by (1→3)-β-D-glucans acquired from a sterilizing cellulose filter. Cytotherapy 2002;4(6):557-9.
- Roslansky PF, Novitsky TJ. Sensitivity of Limulus amebocyte lysate (LAL) to LALreactive glucans. J. Clin. Microbiol. 1991;29:2477-83.
- PDA Technical Report 45. Supplement to PDA J. Pharm. Sci. Technol. 2008; 62: S-2
- Nagasawa K, Yano T, Kitabayashi G, Morimoto H, Yamada Y, Ohata A, Usami M, Horiuchi T. (1→3)-β-D-glucan caused by filtration with cellulose filters in the manufacturing process. J. Artif. Organs. 2003;6:49-54.
- Finkelman, MA, Lempitski, S, Slater, J. β-glucans in standardized allergen extracts. J. Endotox. Res. 2006; 12: 241-245.
- Notarnicola S, Madden, H, Browning J, Coots C, Gunn S, Inman J, Macniven R, Meier W, Podrebarac T, Shepherd A, Wakshull E, Wilson C, Farrington G, Eldredge J, Fure M. ‘Investigation Of A Process Related Contaminant In a Clinical Drug Product’; Biogen, Inc. Cambridge, MA 02142, Recovery of Biological Products XI, Sept 14-19, 2003, Banff Alberta, Canada.
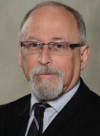
Malcolm Finkelman, PhD (Microbiology & Immunology) serves as the director of Clinical Development at Associates of Cape Cod, Inc. His interests include polysaccharide biology, measurement, and diagnostic assay development. He has worked in the biotechnology industry in process development, regulatory affairs, and in clinical diagnostic development and applications for over 30 years. Dr. Finkelman is currently focused upon (1→3)-β-glucan assay development for fungal infection diagnosis and bioactive contamination control.