MilliporeSigma
The life science business of Merck KGaA, Darmstadt, Germany operates as MilliporeSigma in the U.S. and Canada.
Poor drug solubility has become an increasingly important consideration in the development of orally delivered drugs. For example, although it is reported that 60% of approved APIs are poorly soluble, it is hypothesized that up to 90% of candidates in the development pipeline will fall under this category.1 Therefore, it has become increasingly important to counteract the low bioavailability risks that such poorly soluble compounds can pose. In this area, chemists and formulation scientists have developed a toolkit of strategies that can improve the solubility and subsequent bioavailability of these poorly soluble candidates.2 These approaches include chemical modifications that can be incorporated into synthesis such as salt formation and prodrugs; or formulation modification approaches such as micelle systems, co-solvents, particle size reduction, complexation and amorphous technology.2 Solid-state modification of the API into the more soluble amorphous form is one of the currently preferred methods for enhancing oral bioavailability of these challenging compounds.3,4 Amorphous formulations are especially appealing due to the significant improvement in solubility the amorphous form can provide. By definition, an amorphous solid does not have a long-range order, no crystal structure.2 Due to this, amorphous solids tend to have substantially higher solubilities than their crystalline counterparts as the strength of intermolecular interactions holding the molecules together, and accordingly the energetic barrier to solvation, are lower.2 However, amorphous solids are usually thermodynamically unstable due to the high energy associated with this solid-state form. This instability typically leads to re-crystallization driven by a thermodynamic drive to decrease the energy of the system and return to equilibrium.2-4 It is important, therefore, to develop and optimize an amorphous formulation to ensure sufficient physical stability over the shelf-life of the product. This is an important consideration, as re-crystallization in the formulation would reduce the effectiveness of the formulation for the patient.5 As a result, these stability requirements make an important part of the regulatory dossier required for submission of a new molecular entity, the guidelines of which are outlined in ICH Q1.
To meet these stability requirements, various technologies have been reported. One of the preferred strategies to prevent the recrystallization is to disperse amorphous APIs in a polymeric matrix, referred to as an amorphous solid dispersion.4 The two most common formulation technologies for this application are hot melt extrusion (HME) and spray dried dispersion (SDD). In HME, a mixture of polymer and API is melted and forced through a screw extruder to create a homogeneous mixture.2 This molten mixture is then rapidly cooled and solidified, immobilizing the API within a polymeric matrix which stabilizes the amorphous form. For SDD, the polymer and API are dissolved and co-spray dried, which produces a similar matrix of API dispersed within the polymer.2
In recent years, mesoporous silica has emerged as a formulation option for stabilization and delivery of amorphous API. Mesoporous silica is highly porous silicon dioxide, which can be used similarly to a molecular sponge.2 Upon impregnation of the silica with a concentrated API solution, API is molecularly adsorbed onto the surface of the silica.2 Due to the size of the pores, which have an approximate mean diameter in the nanometer range, the molecularly adsorbed API is locally and sterically confined, preventing re-crystallization2 (Figure 1).
Assessing Amorphous Stability
The inherent instability of an API formulated in the amorphous form was described by Baird et al. as the re-crystallization tendency or “glass forming ability” (GFA).6 Three different GFA classes were defined: class one, drugs recrystallizing upon cooling from the molten state; class two, drugs recrystallizing after a heating-cooling-heating cycle, and class three, drugs that remain amorphous throughout (Table 1).
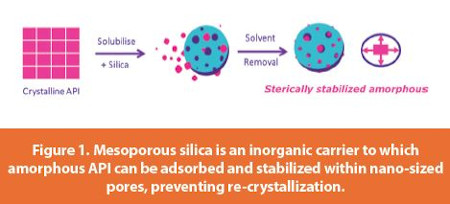
Further work has categorized commercially available pharmaceutical ingredients (API) according to their re-crystallization behavior upon melting.7,8 (Table 1). In these studies, it was described how over 75% of commercially available amorphous formulations contain GFA-3 or “good” glass formers; compounds that are stable in the amorphous form. Contrastingly, only 6% were GFA-1 or “Poor glass formers”. Furthermore, there are only a handful of commercially available GFA-1 compounds that are also BCS II solubility limited compounds. In a recent review, Kawakami provided an overview of pharmaceutical compounds broken down into GFA class.9 Of these compounds, only 29% were determined to be BCS II/IV. This is significant as it is commonly reported that 60% of all commercial compounds fall into this poor solubility category.1 Therefore, there appears to be a disconnect between the rising poor solubility of compounds, and the lack of poorly soluble poor glass formers on the market. This could be related to the difficulty in formulating poor glass formers to meet the regulatory and formulation performance requirements with currently preferred polymeric amorphous solid dispersion technology.
Stability Risks in Polymeric Amorphous Solid Dispersions
Ideally, when an API is dispersed within a polymeric matrix, a homogeneous mixture between the API and the polymer should be obtained, which is referred to as a “solid-solution”.2 However, it is possible for the drug within this polymeric matrix to migrate and form API rich domains, referred to as phase separation. In a phase separated ASD, the drug is more likely to re-crystallize due to close contacts with potential crystal binding partners (Figure 2).10
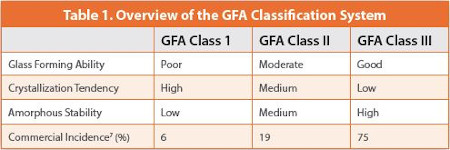
Theoretically, increasing temperature and humidity can affect stability in polymer-based dispersions. As temperature rises, mobility of the drug within the polymeric dispersion is increased. For example, Mehta and co-workers demonstrated that increasing temperature and relative humidity enhanced molecular mobility of all APIs within polymer ASDs, resulting in decreased physical stability of the APIs in the formulation.5 Further work by Mehta and colleagues demonstrated how changes in temperature and relative humidity also had a significant impact on the Johari-Goldstein beta mobility for both celecoxib and indomethacin.5,11 This is an important observation as it has been widely reported that re-crystallization behavior is intrinsically linked with increases in beta relaxation within polymeric matrices. Both of these studies investigated the physical stability of good to moderate glass formers (GFA-II/III), therefore, the effect of moisture and temperature on increasing molecular mobility and subsequent physical instability is likely to be greater for GFA-I compounds with very low glass transition temperatures. This was observed by Ditzinger and Price, who assessed the physical stability of the poor glass formers haloperidol and carbamazepine in HME formulations.12 In their study, it was reported that HME was unsuccessful in stabilizing high concentrations of the two poor glass formers, with only 20% and 7.5% loading successful for carbamazepine and haloperidol, respectively. Furthermore, after the successful formulations were stored under ICH Q1a conditions of 40°C and 75% relative humidity, complete phase separation and re-crystallization was observed after only one week of storage. This instability of the amorphous API within the HME formulations resulted in an inconsistent and decreasing dissolution performance in non-sink biorelevant dissolution. Indeed, by the end of the study, the researchers observed no amorphous solubility advantage in the HME formulations, with the dissolution curve overlapping instead with the unmodified crystalline API.12
Mesoporous Silica: A Potential Solution for Formulating Poor Glass Formers
One emerging approach to successfully formulate GFA-I drugs in the amorphous form is mesoporous silica. Mesoporous silica is able to adsorb molecularly dispersed API inside nano-sized pores, thus stabilizing the amorphous form.2 This approach is of particular interest for GFA-1 compounds due to the high stability that can be achieved by confining drugs in such small environments.2 For example, the work of Muller and co-workers demonstrated amorphous stability at ambient and accelerated conditions for 30 different APIs formulated with mesoporous silica.13
Subscribe to our e-Newsletters
Stay up to date with the latest news, articles, and events. Plus, get special offers
from American Pharmaceutical Review – all delivered right to your inbox! Sign up now!
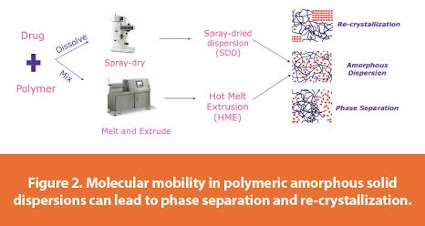
Furthermore, mesoporous silica has been demonstrated to show superior performance in reducing beta relaxation regardless of moisture or temperature. Bras and colleagues demonstrated how nanoconfinement of ibuprofen within mesoporous silica resulted in marked reduction of all known types of molecular mobility.14 Most importantly, the Johari-Goldstein relaxation was significantly reduced, which as previously discussed is related to re-crystallization of the amorphous form.11,14 Similar work demonstrated reduction in molecular mobility and successful stabilization of Menthol (GFA-I). With a glass transition temperature of -54.3°C, menthol is a GFA-1 compound and an extremely poor glass former.15 Nevertheless, the researchers demonstrated a significant decrease in molecular mobility (α and β) when menthol was nanoconfined within mesoporous silica. Furthermore, a new type of molecular mobility, S-type, was observed which was related to hindered mobility and nanoconfinement in the pore, and was significantly slower than standard molecular mobility events.15 Ultimately, the effect of nanoconfinement on this GFA-1 compound resulted in effective stabilization in the amorphous form at room temperature for the duration of the study. For context, a common rule of thumb states that: To be certain a polymeric amorphous solid dispersion is stable, it should be stored at 50° lower than the glass transition.16 In the case of menthol this would require a storage temperature of approximately -110°C, which would not be feasible in a standard formulation development. This pattern of behavior was also observed in the study performed by Ditzinger and Price, who compared the amorphous stability of haloperidol and carbamazepine in mesoporous silica compared to HME. In contrast to the HME formulations studied, mesoporous silica was able to successfully stabilize 30% drug loadings for both haloperidol and carbamazepine. Furthermore, the formulations remained amorphous for the duration of the study, with no evidence of re-crystallization in spite of the high tendency of the APIs to re-crystallize. This translated to a consistent and overlapping dissolution profile in the biorelevant non-sink dissolution tests, with an amorphous solubility advantage preserved throughout.12
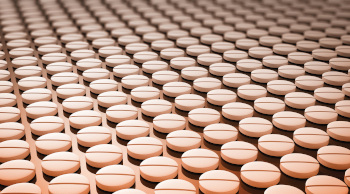
Outlook
Due to the ever-present challenges presented with decreasing solubility in pharmaceutical pipelines, effective technologies to deliver stable amorphous formulations are increasingly important. Although polymer-based approaches have been the technology of choice for decades, there are substantial risks of re-crystallization from polymeric matrices for poorly soluble compounds that are also unstable in the amorphous form, the so-called poor glass formers. This has been demonstrated by a number of theoretical and practical studies that show that molecular mobility of APIs in polymeric matrices can result in re-crystallization. Ultimately, compounds which demonstrate both poor solubility and poor glass stability are not suitable for formulation with typical polymer-based technologies because of this. However, adsorption and nanoconfinement within mesoporous silica has been shown to reduce the re-crystallization risk of these molecules due to a steric and chemical restriction of molecular mobility. Therefore, mesoporous silica is an attractive formulation technology to expand the formulation toolbox for these challenging APIs.
References
- Taylor, LS. and Zhang, GGZ. Physical chemistry of supersaturated solutions and implications for oral absorption. Advanced Drug Delivery Review. 2016; 101: 122-142
- Ditzinger, F. et al. Lipophilicity and hydrophobicity considerations in bio-enabling oral formulations approaches – a PEARRL review. Journal of Pharmacy and Pharmacology. 2019; 71(4): 464-482.
- Hancock, BC. And Parks, M. What is the True Solubility Advantage for Amorphous Pharmaceuticals? Pharmaceutical Research. 2000; 17: 397-404.
- Leuner, C. and Dressman, JB. Improving drug solubility for oral delivery using solid dispersions. European Journal of Pharmaceutics and Biopharmaceutics. 2000; 50(1): 47-60.
- Mehta, M. and Suryanarayanan, R. Accelerated Physical Stability Testing of Amorphous Dispersions. Molecular Pharmaceutics. 2016; 13(8): 2661-2666.
- Baird, JA. et al. A classification system to assess the crystallization tendency of organic molecules from undercooled melts. Journal of Pharmaceutical Sciences. 2010; 99(9): 3787-3806.
- Wyttenbach, N. and Kuentz, M. Glass-forming ability of compounds in marketed amorphous drug products. European Journal of Pharmaceutics and Biopharmaceutics. 2017; 112: 204-208
- Alhalaweh A. et al. Physical stability of drugs after storage above and below the glass transition temperature: Relationship to glass-forming ability. International Journal of Pharmaceutics. 2015; 495(1): 312-317
- Kawakami, K. Crystallization Tendency of Pharmaceutical Glasses: Relevance to Compound Properties, Impact of Formulation Process, and Implications for Design of Amorphous Solid Dispersions. Pharmaceutics. 2019; 11(202).
- Price, DJ. et al. Approaches to increase mechanistic understanding and aid in the selection of precipitation inhibitors for supersaturating formulations – a PEARRL review. Journal of Pharmacy and Pharmacology. 2018; 71(4): 234-281.
- Mehta, M. et al. Effect of Water on Molecular Mobility and Physical Stability of Amorphous Pharmaceuticals. Molecular Pharmaceutics. 2016; 13(4): 1339-1346.
- Ditzinger, F. and Price, DJ. et al. Opportunities for Successful Stabilization of Poor Glass- Forming Drugs: A Stability-Based Comparison of Mesoporous Silica Versus Hot Melt Extrusion Technologies. Pharmaceutics. 2019; 11(11): 22-54.
- Muller RH et al. CapsMorph: 4 years long-term stability of industrially feasible amorphous drug formulations. Int. Symp. Control. Honolulu/Hawai, 2013.
- Bras, R. et al. Influence of Nanoscale Confinement on the Molecular Mobility of Ibuprofen. The Journal of Physical Chemistry C. 2014; 118(25): 13857-13868.
- Cordeiro, T. et al. Stabilizing Unstable Amorphous Menthol through Inclusion in Mesoporous Silica Hosts. Molecular Pharmaceutics. 2017; 14(9): 3164-3177.
- Hancock, C. et al. Molecular Mobility of Amorphous Pharmaceutical Solids Below Their Glass Transition Temperatures. Pharmaceutical Research. 1995; 12: 799-806.