Abstract
Since layer-by-layer (LbL) polyelectrolyte assembly was introduced in the nineteen nineties, it has found application in various fields that study and apply nanotechnology. LbL nanoassembly is based on the sequential adsorption of positively and negatively charged polymers on a surface to form a nano-thick film of coating. The process involves resaturation of polyion adsorption, resulting in the reversal of the terminal surface charge of the film after deposition of each layer. The method provides the possibility of designing ultrathin multilayer films with a precision better than one nanometer of defined molecular composition. These films can be applied to surfaces or can be used to coat micro and nanoparticles. This coating process can be used to control the release of drugs, increase the stability of drugs and to improve the properties of commonly used excipients. Since no covalent binding is involved in the shell formation it allows the drug or excipient to remain intact. Therefore the drug or excipient is not seen as a new chemical entity.
Introduction
In the pharmaceutical industry coating is often used to change and enhance the performance of drug delivery systems, especially solid dosage forms. Traditional coatings include film and sugar coatings. This review will highlight some recent developments in the field of nanocoating as applied in drug delivery. In general there are two types of nanocoatings. These are metallic nanoshells composed of a dielectric core (for example, silica) that is coated with an ultra thin metallic (for example, gold) layer and nano-thick coatings formed by electrostatic layer-by-layer molecular self-assembling (E-LbL or LbL). Both these types of nanocoatings typically have a tiny core (empty or filled) that is sealed in an outer wall of nanometer thickness. For example, gold encased nanoshells have been used to convert light into heat, enabling the destruction of tumors by selective binding to malignant cells. Solid cores, such as nano- and sub-micronized drug particles (typically, 100-1000 nm diameter), can also be encapsulated within a nanoshell. A technique for electrostatic layer-by-layer assembly of organized multilayers through alternate adsorption of oppositely charged linear polyions and protein was introduced in the early 1990s and has been used to form nanoshells around drug and protein cores [1,2]. The basis of the method involves resaturation of polyion adsorption, resulting in the reversal of the terminal surface charge of the film after deposition of each layer. This process in shown schematically in Figure 1.
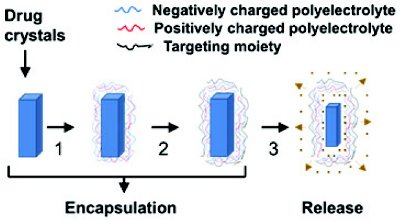
Figure 1. Schematic representation of the electrostatic LbL coating process. This example shows coating of drug crystals for controlled drug release (2). Coating can also be done on fl at surfaces.
The method provides the possibility of designing ultra-thin multilayer films with a defined molecular composition with a precision better than one nanometer. By manipulating the ratio of wall thickness to core diameter and the composition of the wall, the shells can be precisely tuned, to control the release of drugs [3,4]. No covalent binding is involved in the shell formation which allows (macromolecular) drugs and wall colloids to remain intact. The ultimate goal of this wall modification is to achieve a continuous drug release profile, where the drug concentration remains constant throughout the delivery period for hours, and even days. For empty nanocapsules, after thin-film coatings are formed on the templates, cores, the cores can be dissolved to obtain capsules with nanothin walls designed with the desired composition. These empty capsules can be loaded via diffusion, where nanocapsules are suspended in a solution with molecules of interest and concentration gradients drive movement of molecules to the interior of capsules, which are locked in the core as the diffusion gradient dissipates. Controlled release of the capsule load may be reached with pH or temperature changes, or through external physical manipulation, such as IR laser impulse or alternating magnetic field.
In this review, a brief introduction of the preparation and characterization of LbL formed nanocoatings is followed by some examples of how this technology can be used in drug delivery and to improve the properties of drug raw materials and excipients.
Preparation and Characterizing of LbL Coating
The assembly of alternating layers of oppositely charged linear or branched polyions and nanoparticles is simple and provides the means to form 5–500 nm thick films with monolayers of various substances growing in a pre-set sequence on any substrates at a growth step of about 1 nm. These films have a lower molecular order than free-standing films but they have the advantage of high strength and can be easily prepared. For example, a negatively charged material that is a cleaned substrate of any shape and dimension can be immersed into a dilute solution of a cationic polyelectrolyte, for a time optimized for the adsorption of a single monolayer (ca 1 nm thick), and then it is rinsed and dried. The next step is the immersion of the polycation-covered substrate into a dilute dispersion of polyanions or negatively charged nanoparticles (or any other nanosize charged species) also for a time optimized for the adsorption of a monolayer, and then it is rinsed and dried. These operations complete the self-assembly of a polyelectrolyte monolayer and monoparticulate layer double layer onto a substrate as shown in Figure 1.
Table 1. Polyelectrolytes commonly used in LbL assembly of nanocoatings.
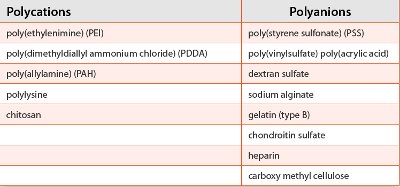
Using this technique, the LbL process allows for nanocoating with linear polycation/polyanion multilayers as well as different nanoparticles, enzymes and proteins in the desired a pre-planned order in a single film on a flat surface or coating a device or particle. The forces between nanoparticles and binder layers govern the spontaneous layer-by-layer self-assembly of these ultrathin films. These forces are primarily electrostatic in nature, but can also involve hydrogen bonding, hydrophobic and other types of interactions. This means that the properties of the self-assembled multilayers depend on the choice of building blocks used and their rational organization and integration along the axis perpendicular to the substrate. For each system studied, the LbL coating can be optimized by varying the polyelectrolytes (Table 1) and fabrication conditions (e.g., ionic strength and pH). In drug delivery the emphasis should be on using non-toxic, biocompatible polymers, including gelatin, chitosan, dextran sulfate, and carboxymethyl cellulose.
A combination of several experimental techniques can be used to study the construction LbL nanocoatings. Multilayer growth can be monitored by UV-VIS spectroscopy that determines cumulative absorption attributed to stepwise deposition of UV- active colloids. Because ellipsometry determines the distance- dependent change in polarization of a light source as a function of reflection or transmission through a substance, it can also be used to measure the multilayer film thickness. In our lab, LbL coating stability is often studied with confocal laser microscopy (CLSM), if fluorescently-labeled polyelectrolytes are assembled in the layers. Although the method is limited to detectable particle size constraints, it is also useful to determine the release of fluorescently- labeled substances from LbL capsules. Multilayer thickness can also be scaled proportionally to the fluorescence intensity.
Quartz crystal microbalance studies, which relate vibrational dissipation (QCM-D) as function of the step-wise amount of a colloid adsorbed to a quartz crystal surface in real time, is also used to determine the thickness of LbL nanocoatings. These coatings are usually done on QCM crystals and the results are then extrapolated to estimate the thickness of coatings on the material of interest such as a drug crystal. Since LbL coating changes the surface charge of drug particles, zeta potential measurements (determined by electrophoretic mobility) can indicate the efficiency of charge reversal by alternating colloids, therefore also the efficiency of the coating process. Examples of results obtained by CLSM, QCM and zeta-potential measurements are shown in Figure 2.
These are some of the more common techniques that are currently used to confirm the self- assembled LbL process when coating drugs and drug delivery devices.
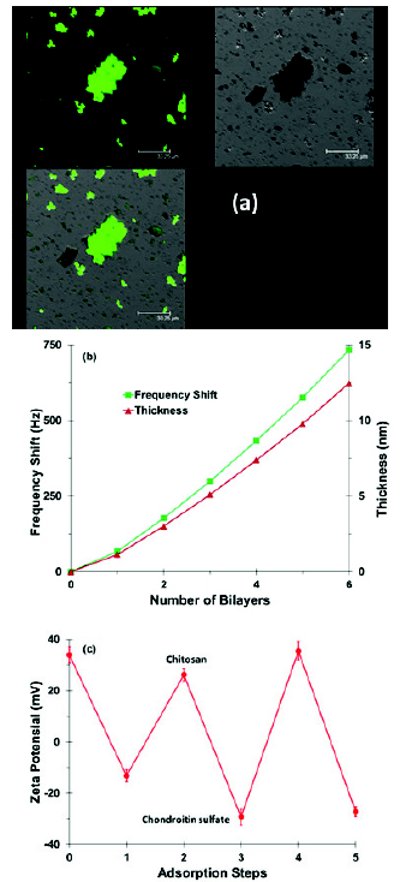
Figure 2. (a) CLSM picture of LbL coated nifedipine drug crystals. Fluorescence comes from FTIC labeled chitosan used in the coating process. (b) QCM data showing the increase in film thickness after the sequential absorption of chitosan and chondroitin sulfate onto a QCM crystal. (c) Zeta potential data showing the sequential absorption of chitosan and chondroitin sulfate onto nifedipine crystals (notice the reversal of charge after each coating). These are some of the more common techniques that are currently used to confirm the self-assembled LbL process when coating drugs and drug delivery devices.
LbL nanocoatings offer several advantages to other methods of encapsulation, coating or fixation of used in drug delivery and formulation:
- the coating thickness can be tailored in the nm-μm range
- several types of synthetic/natural polymers and nanoparticles can be used for LbL coating
- the location and sequence of the nano- thick layers can be controlled
- surface labeling with targeting molecules is possible
- stabilization and surface protection of submicron particles is possible
- LbL coating eases the use of thermodynamically unstable mechanically-micronized particles
- compared to a minimum of ~10% of colloids and/or polymers needed with conventional coating techniques, much smaller amounts of colloids polymers (1 %) is needed to produce a functional LbL assembled coating.
Historically it can be said that the first ten years of LbL self-assembly laid the physicochemical foundation for the manufacturing of LbL constructs and the characterization thereof. During this period, different substances were also loaded into and released from these systems dependent on coating architecture and stimuli. Since 2000, LbL drug delivery systems have rapidly developed. The robust nature of the self-assembly process, the high encapsulation efficiency, targeting and biocompatibility of LbL coated systems holds great potential for controlled and targeted release of drugs and genes.
One well-established application of LbL coating is to control the release of drug from coated micro- or nanoparticles. For example furosemide microcrystals were nanoencapsulated by gelatin/PSS/ PDDA multilayers [3]. This coating prolonged drug release by 50- 300 times based on just 2 or 6 bilayers respectively which reached a maximum thickness of ~ 155 nm. In addition the layers can be designed to release the drug based on environmental changes (pH, temperature and ionic strength) or external stimuli (heat, infrared irradiation, magnetic forces, etc.). In Figure 3 the drug release from spray dried microspheres coated with a pH sensitive coating is shown.
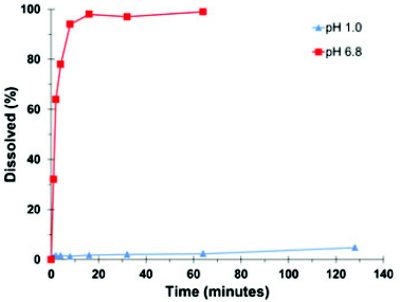
Figure 3. Controlled drug release from furosemide spheres (~ 10 μm) produced by spray drying and LbL coated with a pH sensitive coating. Less than 5 % drug is released in 2 hours at pH 1.0 compared to almost 100 % within 20 minutes at pH 6.8.
In addition to controlling the release of drug from nanoparticles by LbL coating, recent studies have shown that LbL coating can potentially eliminate the instabilities associated with many drug nanoparticle dispersions because in contrast to surfactant stabilizers, LbL coatings do not detach easily from the surface and retain integrity upon dilution/reconstitution in other media.
This shell can therefore be designed to improve the stability of drug nanoparticles in suspension. Furthermore, the inner layers minimize the surface free energy of the encapsulated, small core particles, thereby preventing crystal form changes and nanoparticles coalescence, whilst the outermost layers due to their high hydrophilicity and strong surface charge enhance colloidal stability [4,5]. Intermediate layers in the shells that tie the multilayer together can be designed to serve as a dissolution barrier thereby allowing modification of and control over the release of the drug from the nanoparticles. These are just a few examples of the potential of LbL coating in drug delivery. In our laboratories we have also used LbL coating to produce directly compressible excipients and drug particles, and to protect drug and excipient particles against moisture and light.
In the future we anticipate that the array of colloids and polymers (synthetic and biological) utilized in LbL assembly will become more sophisticated. Polyelectrolytes might assume hybrid forms through functionalization of monomers with drug, gene or nucleic acids and other moieties preceding polymerization. These hybrid colloids will perform simultaneous roles as structural constituents, drug carriers/prodrugs and stimuli-sensitive release triggers. LbL stabilization will also be used to improve otherwise troublesome, unstable carriers such as liposomes or micelles. The coatings will show more specific stimuli-responsiveness as was already shown for glucose-specific enzyme multilayer systems [6]. It can be imagined that LbL reservoirs, containing therapeutic agents, could be implanted in patients with a genetic predisposition to detrimental conditions. When disease markers are encountered by these implanted LbL capsules, a therapeutic agent will be released. Such systems are envisioned for long-term treatment with biodegradable LbL coated implants containing drugs useful in treatment of tumors. These implants could be situated close to or inside the tumor, ensuring long-term sustained release of the drug. The same principle could probably be applied to the delivery of genes/DNA fragments to patients with metabolic defects to ensure long-term treatment.
Conclusion
LbL self-assembly systems are very promising and could provide a very robust platform to create novel, hybrid or stabilized drug delivery systems for a myriad of drugs and genes. The technology can be employed on various nano- to macroscopically-sized systems. Due to the technology’s ease and cost of preparation, avoidance of hazardous preparative chemicals, independence of precise stoichiometry, facilitation of controlled, triggered and target release, the technique should see significant growth in the future as a leading drug delivery technology. Concurrently to developments in drug delivery, LbL nanocoatings has also found numerous applications in improving the stability and processing of drug solids and excipients.
References
- G.B. Sukhorukov, H. Möhwald, G. Decher, Y.M. Lvov, Assembly of polyelectrolyte films by consecutively alternating adsorption of polynucleotides and polycations, Thin Solid Films, 284-285 (1996) 220-223.
- M.M. de Villiers, D.P. Otto, S.J. Strydom, Y.M. Lvov. Introduction to nanocoatings produced by layer-by-layer (LbL) self-assembly. Adv, Drug Deliv. Rev. iews.
- H. Ai, S.A. Jones, M.M. de Villiers, Y.M. Lvov, Nano-encapsulation of furosemide microcrystal for controlled release, J. Controlled Release. 86 (2003) 59-68.
- N. Pargaonkar, Y.M. Lvov, N. Li, J.H. Steenekamp, M.M. de Villiers, Controlled release of dexamthasone from microcapsules produced by polyelectrolyte layer-by-layer nanoassembly, Pharm. Res. 22 (2005) 826-835.
- T. Wu, Y. Sun, N. Li, M.M. de Villiers, L. Yu. Inhibiting surface crystallization of amorphous indomethacin by nanocoating, Langmuir, 2007, 23, 5148-5153.
- D. Lee & T. Cui. Low-cost, transparent, and flexible single-walled carbon nanotube nanocomposite based ion-sensitive field-effect transistors for pH/glucose sensing, Biosens. Bioelectron. 25 (2010) 2259-2264.
Author Biographies
Yuri M. Lvov, Ph.D., is a Professor of Chemistry and T. Pipes Endowed Chair on Micro and Nanosystems at the Institute for Micromanufacturing, Louisiana Tech University. Lvov was among pioneers of the polyelectrolyte layer-by-layer assembly which became an important method in bio/nanotechnology. His research on LbL assembly has been cited more than 9,700 times. In 2008, he received the Best of Small Tech US National Innovator Award in recognition of his achievements in the field of nanotechnology.
Daniel P. Otto, Ph.D., is a Post-Doctoral Researcher from the Catalysis and Synthesis Research Group (CRB Focus Area) at the North-West University, Potchefstroom, South Africa. He completed part of his post-doctoral study working at the School of Pharmacy at the University of Wisconsin. His research interests are in general nanotechnological pharmaceutics and in particular designing drug delivery systems for controlled and targeted delivery using LbL self-assembly. He is the co-author of 10 papers in the field of nanosized drug delivery systems.
Melgardt M. De Villiers, Ph.D., is a Professor at the University of Wisconsin School of Pharmacy. His current research is focused on determining the pharmaceutical science involved in developing an understanding of the pharmaceutics, engineering, and materials sciences principles underlying enhanced drug delivery technologies. Several of his publications have been related to layer-by-layer nanocoating and to solid-state properties of drugs and excipients. Dr. De Villiers is the associate editor of AAPS PharmSciTech and the recipient of the Outstanding Professor in the College of Health Sciences at the University of Louisiana at Monroe.