Introduction
A multipronged approach is used to ensure the safety of mammalian cell-derived biotechnology therapeutics from adventitious contaminants such as viruses and mycoplasma. Extensive characterization and testing of the cell substrate, lotto- lot release testing for adventitious contaminants and viral clearance characterization of the downstream purification unit operations are integral parts of this approach. Adherence to current Good Manufacturing Practices (cGMP) and a robust raw material quality review form the cornerstones of this safety approach [1-5]. Consequently, there have been no reported cases of transmission of an adventitious agent through biotechnology derived therapeutics and as such these products have an untarnished safety record. Although very rare events, contaminations of mammalian cell culture bioreactors with adventitious agents have been reported [6-11] and present a business continuity risk. To further safeguard against such contamination events, technologies capable of inactivating adventitious agents that may be introduced through raw materials could be implemented. The desired technologies should act as barriers to the introduction of adventitious contaminants into the cell culture process, thereby mitigating the risks of a contamination. Such technologies should also not impact the cell culture performance, downstream process performance or product quality.
In a proactive approach to risk management, we have studied the susceptibility of two relevant model viruses to inactivation in cell culture media by the treatment with two distinct inactivation technologies. These include high temperature, short time (HTST) pasteurization and ultraviolet light in the C spectrum (UV-C). These model viruses were chosen because they have been reported as contaminants of Chinese hamster ovary (CHO) cells [7-9]. CHO cells are also a widely used cell substrate in the biopharmaceutical industry for production of therapeutic proteins. This makes understanding the inactivation of potential viral contaminants in CHO cells particularly important.
The focus of the studies summarized in this report was limited to evaluation of the virucidal efficacy of HTST and UV-C. Each technology is currently used in various industrial settings due to their virucidal efficacy and risk mitigation potential. In HTST treatment, fluids such as media are exposed to elevated temperature for short duration (seconds to minutes) to inactivate potential contaminating microbes and rapidly cooled to reduce component degradation. HTST is used primarily in the food industry for continuous-flow pasteurization, but it is also being used in the biopharmaceutical industry [7]. Several lines of research have demonstrated robust inactivation of virus and mycoplasma in milk upon treatment with HTST [12-14]. In HTST-treated milk, a 4-log reduction in foot-and- mouth disease virus (FMDV) [12] and a 4-5.2 log reduction in Mycoplasma avium titer have been observed [13].
UV-C is another technology known to have robust virus inactivating capabilities. UV-C, treatment involves exposing a fluid of interest to UV-C light before use. Increasingly, UV-C is being used to inactivate potential viral and bacterial contaminants in donated blood, cell culture media, and liquid food, often replacing traditional heat pasteurization [15-16]. UV-C is a newer technology in contrast to heat pasteurization, which has been in use for over 150 years. UV-C has been used since 1949 in the manufacture of biologicals, in particular blood- and plasma-derived products, for the purpose of inactivating viral contaminants [17]. Significant log reductions have been reported for viruses (4-8 logs) and various mycoplasma species (>7 logs) upon UV-C treatment of virus or mycoplasma spiked fetal calf serum [18].
Data from these studies show that both HTST and UV-C treatment can robustly inactivate the challenge viruses. These methods can be used as effective upstream barriers to the introduction of adventitious agents such as viruses and mycoplasma.
Experimental
Two model viruses, mouse minute virus (MMV) and Cache Valley virus (CVV) were chosen because they have been reported as contaminants of CHO cell cultures. Additionally, they represent extremes of a physico-chemical resistance spectrum. Mouse minute virus is a member of the autonomously replicating parvoviridae family of viruses, which are classically characterized as icosahedral, non-enveloped, single-stranded DNA viruses with approximate sizes of 20 to 25nm [19]. MMV is a lytic virus that thrives specifically on proliferating cells where cell death usually results once the virus life cycle is complete. MMV is capable of active proliferation in CHO cells and is commonly used as a model virus for reduction studies during the assessment of the viral clearance potential of various purification process unit operations in the biopharmaceutical industry. MMV particles are highly stable and resistant to many commonly employed inactivation methods such as low pH, heat, exposure to high salt concentrations and extraction with lipid solvents [20]. This resilience is primarily due to lack of a lipid envelope which, in contrast, enables the relatively easy inactivation of enveloped viruses such as CVV using the same methods. As such, MMV served as a worst case model for HTST treatment. Because MMV can survive desiccation, it may potentially be introduced through a number of raw materials used in cell culture processes.
CVV is an enveloped, single-stranded, negative-sense RNA virus and is about 4.5 kilobases (Kb). CVV’s lipid, bilayered envelope is about 5 to 7nm thick, and the virus is between 80 to 120nm in diameter. It is a member of the Bunyaviridae family of viruses [21]. CVV, like MMV, is of concern to the biopharmaceutical industry because it can infect CHO cells and therefore poses a threat to the cell culture. The most likely route of entry into a manufacturing facility to cause such an infection is through raw materials of bovine origin, such as serum [9].
The general experimental design for the evaluation of the virucidal efficacy of HTST or UV-C is described in Figure 1. A spike-treat culture experimental approach was designed. In these studies, each of three cell culture media types (1, 2, or 3) was spiked with MMV or CVV and treated with either HTST at 102.0°C for 10 seconds or with UV-C at a fluency of 125 mJ/cm2. Fluency is a function of the intensity of radiation multiplied by the residence time, or duration of exposure to UV light. The virus-spiked, treated media was then used to culture three different recombinant CHO cell lines (A, B or C -one for each media type) under conditions intended to support cell growth and virus replication. The resulting cell cultures were assayed for relative increase in virus genome copy number over time using virus specific real time PCR (qPCR) assays. This spike-treat-culture approached allowed the testing of a large portion of each treated media type as well as in situ amplification of any residual infectious virus particles remaining after each treatment regime. This approach significantly increased the sensitivity of detection of any residual infectious virus particles remaining after each treatment regime.
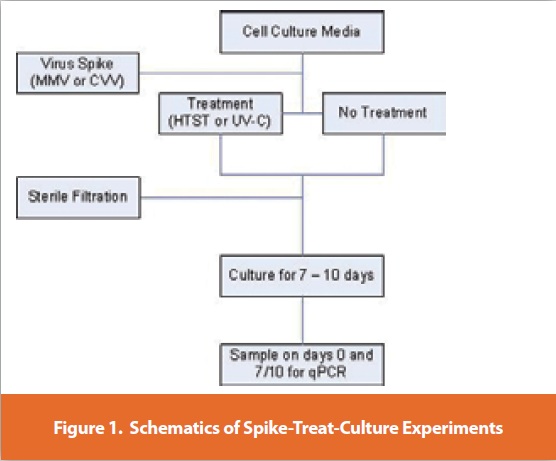
After each treatment of spiked media, the HTST or UV-C unit was chemically sanitized and media without virus (unspiked) was then processed through either instrument without heat or UV-C light (not depicted in Figure 1). This media sample served as the negative control (NC) and allowed for the simultaneous qualification of the cleaning procedure. Aliquots of each negative control sample were subsequently sterile filtered using a 0.22 μm filter and stored at 4°C to 8°C prior to use in cell culture. A third media aliquot was spiked with a known quantity of each virus and was left untreated to serve as a positive control (PC). The two controls and the virus-spiked, treated media were then used to grow three recombinant CHO cell lines. The cells were observed over 7 to 10 days for signs of viral infection.
HTST treatment of each medium type was carried out at a temperature set point of 102.0°C and a residence time of 10 seconds. In order to achieve the target UV-C fluency rate, treatment of the various media required modulation of the flow rate in order to compensate for differences in optical absorbance at 254 nm. Subsequent to treatment, aliquots of each treated media were sterile filtered using a 0.22 μm filter and stored at 4°C to 8°C.
The treated and control samples, for each media type, were used to culture the appropriate cell line (cell lines A, B or C) for 7 to 10 days. Samples were assayed for increase in viral genome copy number using virus specific qPCR assay. Known quantities of input virus in the treated samples and positive control samples were used to calculate a minimum log reduction value attributed to each treatment regime.
The results demonstrate that both treatment technologies provide an effective means of virus inactivation. The log reduction values calculated and reported in this report were limited by the titer of the input challenge virus and the necessary dilution of the challenge virus in media as well as the titer of the virus in the positive control sample. As such, the values reported here are considered to be minimum log reduction values. Both enveloped and nonenveloped model viruses were effectively inactivated and no virus amplified in the culture phase of the spike-treat-culture approach. The use of highly susceptible cell lines cultured with large volumes of the treated media significantly increased the confidence in the viral inactivation profile observed for the two technologies tested.
Conclusions
Ensuring the safety of biotechnology derived therapeutics produced in mammalian cells is achieved through multiple layers of control from the cell substrate through the purification process. Studies have demonstrated that cell substrates such as CHO do not have a wide range of susceptibilities to viruses [22]. In addition, those viruses that are capable of infecting and proliferating in CHO cells are detected during the lot-to-lot quality control tests or through observed changes in cell culture parameters [22]. Adventitious contaminations of cell culture, with viruses and mycoplasma, have nevertheless been reported. The most likely route of entry for such contaminants has been postulated to be raw materials such as media, serum or other cell culture additives. Therefore, it is important to explore technologies that create barriers to the introduction of such adventitious contaminants. We evaluated two technologies for treatment of cell culture media and demonstrated that both technologies are effective for inactivation of viruses. Two model viruses at the extremes of the physico-chemical susceptibility spectrum were utilized for these studies thus allowing extrapolation of the inactivation data to other virus and mycoplasma species within this range. Although the focus of the studies presented here was virucidal efficacy of these technologies, any potential impact of such treatment regimes on cell culture processes and product quality should be extensively evaluated.
References
1. ILL, C.R.; Dehghani, H. Curr Opin Drug Discov Devel 2009, 12 (2), 296-304.
2. Ruffing, M. American Pharmaceutical Review. September/October 2009. 35-38.
3. ICH. Q5A. International Conference on Harmonisation of Technical Requirements for the Registration of Pharmaceuticals for Human Use: Geneva, Switzerland. 1998.
4. EMEA. EMEA/CHMP/BWP/398498/2005. European Medicines Agency: London, 2008.
5. FDA. Points to Consider in the Manufacture and Testing of Monoclonal Antibody Products for Human Use. Bethesda, Maryland. 1997.
6. Dehghani, H.;et al. Proceedings from the PDA Workshop on Mycoplasma Contamination by Plant Peptones, Potts, B.J., Ed. 2007, 53-59.
7. Garnick, R.L. Dev Biol Stand. 1996. 88:49-56.
8. Garnick, R.L. Dev Biol Stand. 1998. 93: 21-29.
9. Nims RW. Developmental Biology. 2006. 123:153-164.
10. Genzyme. 2009. http://www.genzyme.com/corp/media/GENZ%20PR-061609.asp
11. Oehmig, A. et al. J Gen Virol. 2003. 84: 2837-2845.
12. Tomasula, P.M., et al. Journal of Dairy Science. 2007. 90(7):3202-3211.
13. Grant, I.R., et al. Applied and Envrionmental Microbiology. 2005. 71(6):2853-2861.
14. Terpstra, F.G., et al. Breastfeeding Medicine. 2007. 2(1):27-33.
15. Sofer, G. BioPharm International. 2003. S30-S33.
16. Caillet-Fauquet, P., et al. Journal of Virological Methods. 2004. 118:131-139.
17. Wang, J., et al. Vox Sanguinis. 2004. 86:230-238.
18. Kurth, J., et al. Dev Biol Stand. 1999. 99: 111-118.
19. Cotmore, S.F., Tattersall, P. Advances in Virus Research. 1987. 33: 91–174.
20. Siegl, G., et al. Intervirology. 1985. 23(2):61–73.
21. Schmaljohn, C., Hooper, J.W. Fields virology, 4th ed., Philadelphia: Lippincott Williams & Wilkins; 2001, p. 1581-1602.
22. Berting, A., et al. Biotechnology and Bioengineering. 2010. 106: 598-607.
Author Biographies
Dr. Houman Dehghani is a Principal Scientist at Amgen, Inc. His area of expertise is viral and microbial safety evaluation of mammalian cell culture processes. Currently he leads the Biosafety Development Laboratory at Amgen, which is involved with development and assessment of new technologies for detection and identification of adventitious contaminants of cell culture, and viral clearance assessment studies in both cGMP and development settings. He holds a Ph.D. degree from Michigan State University.
Marc Schleh holds a B.S. (2004) in Molecular, Cellular and Developmental Biology from the University of Washington. As a Senior Associate at Amgen, Marc was involved in assessment of new technologies for inactivation of viruses. Currently, Marc is pursuing a Master’s degree at Georgetown University.
Bill Lawrence is a Scientist with Amgen’s Biosafety Development Group specializing in design and implementation of quantitative PCR assays as well as a variety of other molecular biological techniques. During his ten years at Amgen Bill has used his skills for gene discovery efforts, biomarker analysis, and most recently in the Biosafety Development Laboratory to evaluate new technologies for detection and identification of adventitious contaminants of mammalian cell culture processes.
Tom Park joined Amgen 9 years ago, performing cell culture operations in the Process and Development Facility. In his current role as a Senior Engineer in the Global Process Engineering group, Tom provides commercial process development, technology transfer, and process characterization support for various projects. Tom has extensive cell culture and process modeling expertise. Tom holds a B.Sc. degree in Biological Engineering Oregon State University.
Scott Rosenthal, Ph.D. holds a B.S. (1989) in Chemistry from Western Michigan University and a Ph.D. (1995) in Biochemistry from Purdue University. He has over 15 years of experience in academia and industry focusing on protein purification and the application of viral clearance strategies to protein therapeutic processes. Prior to joining Amgen’s Purification Process Development group as a Senior Scientist, Scott worked at Bayer’s Biological Products division in the Pathogen Safety and Process Development groups.
Roger Hart, Ph.D. obtained a B.S. in Biomedical Engineering from University of New Mexico in 1985 and a Ph.D. in Chemical Engineering from California Institute of Technology in 1991. Prior to Amgen, he worked at Genentech in the Purification Process Development department. Between 1996 and 2006, he led a research group in early Process Development at Amgen focused on advancing products and technologies. Since 2006, he has been a scientific director in late stage Process Development at Amgen.