Introduction
Drug efficacy and safety have traditionally been considered as predicated by dose, its frequency and the duration of drug or active metabolite at the site of activity. Such variables are usually explored in investigative clinical trials prior to pivotal studies. It is increasingly apparent however that many additional influences can determine drug effects. Traditional clinical assessment programs can accordingly, and with hindsight be considered rudimentary. “Trial and error” was essentially the modus operandi. Simplistically “the wrong patient could be taking the wrong amount(s) of drug at the wrong time” (delivered to the wrong place). Such practices could also explain, to some degree the limited effectiveness of some current medications [1 and Table 1].
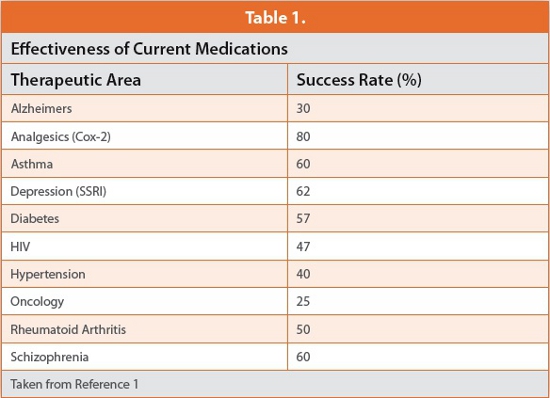
Medications were also invariably designed as a bolus for “immediate release” on ingestion or administration. Dosage forms incorporating some form of controlled release were usually only developed when extensive, post-approval experience highlighted nuances of drug action that merited dosage form redesign.
The present situation, where new drug development programs can be slow, expensive and failure-prone could be grounds for pessimism. However, there are many reasons to be optimistic concerning possibilities for novel drugs, not least because of the following considerations:
• Increasing awareness, and knowledge of the role that an individual’s genetic makeup can play in the absorption, disposition and metabolism, as well as the pharmacologicalactivity of a drug. Knowledge of pharmacogenetic and other “person-specific” effects can help select (or de-select) patients, whose genetic makeup or other attributes make them suitable or otherwise for a particular medication.
• The presence or levels of many endogenous substances may reflect susceptibility to or state of a clinical condition, its progression or alleviation. Interest and activity in such biomarkers has burgeoned due to their potential to provide more reliable and earlier readouts of clinical efficacy, drug toxicity, or as molecular markers for early disease detection. Some may, in time become surrogate indicators for efficacy and safety.
These and other advances should help and expedite the quest for novel, safe and effective medications. If promise is to become reality however, more sophisticated drug delivery than bolus dosage will be required. There will need to be greater alignment of delivery with the dynamics of drug action, with the patient and with the state of the clinical condition. It will also be necessary to design, execute and interpret more imaginative “proof of concept” or other early clinical assessments to identify optimal drug delivery modes. This article reviews the rationale, challenges and possibilities for such better dosage form design and offers near-term and future perspectives on controlling drug delivery.
Controlling Drug Release to Enhance Performance
The ideal drug delivery system provides the right amount of drug or active metabolite to the target at a time and rate that optimizes its effect without compromising safety. Controlling release to meet these requirements can involve preventing release or delivery in enzymatically or other hostile environments, targeting a specific location to enhance therapeutic index, or prolonging delivery to sustain an eff ect. “Delivery on demand” may also be required.
Dose (Right Amount)
Most medications are provided in a limited number of doses. However, it has become increasingly evident that there can be significant interpersonal differences in how a drug is absorbed, distributed, metabolized and cleared. The genotype of the patient can have a major influence, not only on these factors but also on the intrinsic pharmacology of some medications. Non-genomic influences include age, body mass, lifestyle, nutrition, the state of the clinical condition, and whether the patient has other conditions, or is taking other medications. Such complexity means that dose, and possibly rate of input may need to be individualized for each patient for optimum effect. Capability to rapidly alter dose if warranted by biomarker measurement or other clinical signal may also become the norm as companion diagnostics become widely available, reliable and easy to use. Therapy would thus be optimized for the individual patient (Table 2).
Personalized Medication will probably require that a range of doses units be available in convenient form. This has implications for dosage form design and also for manufacture. Current “Fordism”-based paradigms of limited product variants and economies of scale may no longer be tenable.
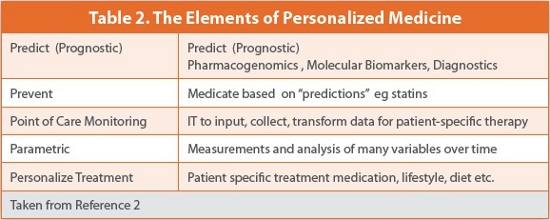
Right Place (Drug Targeting)
Delivery to the site of drug activity, be it organ, tissue or cell can optimize effect and reduce side effects. Drugs such as antibacterials have been incorporated in bone cement in hip or knee replacement surgery, as have anti-clotting agents in cardiac stents and angioplasty balloons. Rates of elution from such systems to evince the required “local” effect need to be controlled appropriately. The nature of drugs used in cancer treatment is usually such that site specific targeting is virtually mandatory, to avoid collateral damage to healthy cells. This is conceptually possible if the cancer is located in a specific tissue or organ. Particulate compositions, recognized by the reticulo-enothethial system as “foreign”, and accordingly concentrated at locations such as lymph nodes, liver and spleen have been investigated and, in some cases commercialized. “Homing devices” include polymeric micelles nanoparticulate materials or liposomes. Magnetically responsive particles in the dose have also been assessed, the composition being guided to and localized at the target site by an external magnetic field [3]. Such strategies might also pertain to other localized conditions like some inflammatory diseases or infections but no applications have been reported.
“Right-place-delivery” can also mean delivering drug to the lower intestine (colon) to treat conditions such as Inflammatory Bowel Disease (IBD). Release in the upper gastro intestinal tract is prevented using suitable coating materials, to avoid systemic absorption and conserve drug for local effects in the colon. However, the colon is not well designed for systemic absorption. The semi-solid and relatively static contents prevents drug spreading and migration to the colonic wall for absorption. Colonic surface area for absorption is also much less than in the upper intestine. Nevertheless, several drugs have been shown to be absorbed from the colon (Table 3) [4].
Colonic delivery has also been considered for delivery of proteins or peptides to the systemic system, by protecting them from peptidases in the upper intestine, while en route to the colon. However, there has been limited success to date although efforts continue.
Where a condition may prevail throughout the intestines, as with microbial or protozoal infections it should be possible to sustain drug presence by using coating polymers that are soluble at different pH values, drug being released at various intestinal locations as pH changes. Such technology is reliable and has stood the test of time so there are no obvious needs for innovations for such delivery.
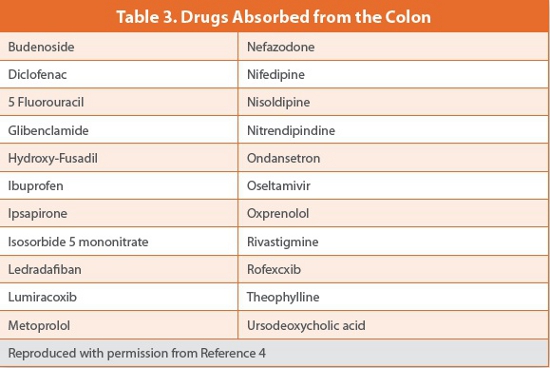
Delivering drugs to the Central Nervous System, particularly the brain is challenging as the blood-brain barrier presents formidable hurdles. Alzheimer’s Disease is associated with brain insulin deficiency/resistance. Hence, delivery of insulin to the brain could provide therapeutic benefit. However parenterally administered insulin causes hypoglycemia in non-diabetics. A recent investigative study has shown that intranasal administration directs insulin to the brain, avoiding systemic side effects. Dosing resulted in significant improvements in learning, memory and cognition, contrasting with continued deterioration in control subjects [5]. Such innovative targeting illustrates how mode of delivery may, on occasion, direct the drug to a specific location. A combination of knowledge of routes of drug entry to the systemic compartment, mode of transport, target location as well as the molecular biology of a clinical condition can identify possibilities for new ways of controlling drug delivery.
Intracellular Delivery
The locus for activity of most physiological modulators is intracellular: Drugs are no exception but barriers to cell entry can be formidable and the intracellular milieu can be hostile to “foreign” entities. Systems are highly primed for ready expulsion or transformation of unwanted agents. Concepts being envisioned to overcome such barriers include capitalizing on modes of cell recognition and transport to gain entry. The most creative efforts concern oncology drugs. Solid tumors in particular are resistant to penetration by drugs. It is widely recognized that effective cancer chemotherapy requires a smart delivery system that is able to survive metabolic assault, surmount barriers and possess the requisite homing capability to attack and destroy the cancer cells, leaving others unharmed.
Nano robots (nanobots) are exciting great interest for use with diagnostics but also for site specific drug delivery. One approach concerns structures assembled from DNA. DNA nanobots possess valuable “design capability”. A long single strand of DNA can be coupled with short strand fragments to provide a “DNA cage” of requisite size and shape. Such “clam-shell-like” structures can exist in open or closed form due to the presence of “DNA hinges” (Figure 1 (i)). The closed shell, in conjunction with anchor strands within the cage and complimentary DNA linker strands attached to the drug secure its retention (Figure 1 (ii)). The cage is locked by DNA double helices.
The DNA nanorobot surface can be designed to “recognize” only cancer cells while a cancer specific protein within the cell “unlocks” the cage to release drug. Such “trojan horse” constructs have been shown (in vitro) to target a series of six different cancer cell lines. Payloads of antibody fragments in the cage consistently induced cell apoptosis [6]. Possible issues with such delivery might concern whether, on cage opening “freed” cytotoxics enter nearby healthy cells by diffusion or following cancer cell apoptosis’.
Such inventive approaches approaches could also conceivably provide “platform technologies” for intracellular targeting of other therapeutic agents.
Chronotherapeutic Considerations (Right Time)
Some clinical conditions have “time” components that influence the effects of medications: Asthma is a well-known example, attacks peaking in early morning, particularly in childhood (Figure 2). Hence, maintaining constant theophylline levels throughout the day is less beneficial than dosing to provide “peaks and troughs” at the appropriate times in the circadian cycle [7].
Many cardiovascular conditions have time-related components. The onset of myocardial infarction is highest in the morning, as are sudden cardiac death, stroke, ventricular arrhythmias and arterial embolism. Angina attacks are also more frequent in early morning. [8,9]. It is surprising that anti-hypertensives are often prescribed for morning dosing, possibly reflecting practices in their pre-approval clinical development programs and patient compliance considerations [10 and Table 4]. Morning dosing may be sub-optimal and some antihypertensive agents have now been formulated in delayed/controlled release forms to deliver drug, prior to morning rising.
Peptic ulcer disease is generally lower in Summer and peaks in Autumn [11]. Duodenal ulcer rates have also shown a 6-month rhythm, peaks occurring in May-June and in Nov-Dec [12]. There may be opportunities for seasonal prophylactic treatment for these conditions.
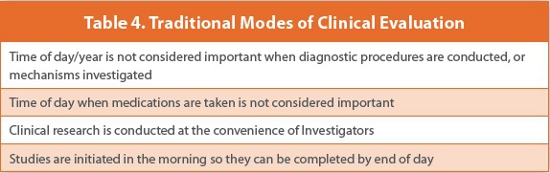
“Right time” may involve keeping drug at the site of activity for the requisite time to initiate or sustain an effect. There are many technologies for sustaining drug presence in the gastro intestinal tract to prolong absorption and extend presence in the systemic system, or to minimize plasma peak/trough ratios and associated side effects. However, oral dosage can be an unreliable route to the systemic system for controlled release dosage forms. The gastro intestinal milieu is not consistent nor are transit rates, especially in the stomach. Small intestinal transit time is also relatively short (about three hours), leaving limited time for drug absorption, particularly where absorption is limited to the upper small intestine. Additionally, metabolizing and recycling enzymes in intestinal epithelium can reduce or lead to inconsistent absorption. Gastro retentive systems that purport to retain the drug or dosage form in the stomach in order to sustain drug presence in the small intestine to prolong absorption have not been very effective [13]. Attempts to deliver proteins or large peptides via the oral route have also, with a few exceptions been unsuccessful. However, for drugs with suitable physico chemical properties, dose response and mode of action the oral route is likely to be first choice, as the prevalence of many products can attest.
Delivery on Demand
The ideal delivery system delivers drug “when required”, like many endogenous mediators. Current “delivery on demand” practices are essentially manual, such as drug being inhaled in acute asthma or insulin injected following blood glucose readings. Futuristic strategies envision more sophisticated delivery for a wide range of indications. Systems might comprise a biosensor linked to a delivery device/drug reservoir. The information provided by the sensor is translated to a dose (“instruction”) to the delivery device, which delivers the requisite amount from the reservoir. The biosensor is ideally located within the systemic system for optimum sensitivity. A system for transdermal monitoring of glucose levels is currently in development, with potential to continuously record and communicate data to a remote wireless device [14]. This could conceptually be linked to a delivery device to provide the requisite amount of insulin “on demand”.
A delivery device, external to the systemic system would seem most appropriate in terms of non-invasiveness and replacement/ replenishment. At the same time, more effective targeting and control is more likely if monitoring and delivery occur within or close to the target organ or tissue. Nanotechnology could enable the construction of nanorobots providing scanning, sensing and signaling capability within systemic compartments. Reduction of such concepts to practice may take some time but possibilities are being envisioned.
Diagnostics and Evaluation Programs
Biomarkers
With the exception of anti infective agents, where the microbe is essentially the “receptor”, and some acute short-term ailments the establishment and definition of an optimum target drug plasma or tissue profile prior to clinical trials has been difficult, time consuming and error-prone. There was little option but to establish a maximum tolerated dose, estimate dose frequency based on systemic clearance or in vitro receptor occupancy, and proceed to proof of concept studies. Biomarkers have potential to surmount such roadblocks. New putative biomarkers are being identified virtually daily in oncology, cardiovascular diseases, Parkinsonism, Alzheimers and many more. Biomarker tests are already playing a major role in studies seeking to provide personalized therapy in oncology in predicting tumor response and resistance to chemotherapeutic agents. Diagnostic kits are increasingly available. 2011 saw a first approval by FDA of a drug, along with a companion diagnostic for treating melanoma [15].
Many biomarkers may flatter to deceive and it may be necessary to capture and interpret values from several arrays of such agents and translate to useful information. Nevertheless, their potential as data-based indicators of a clinical condition and of the influence of a drug being used in its treatment could help determine the effect of different drug plasma profiles on clinical response in preclinical or early clinical studies. Biomarkers have the potential to transform dosage form design programs to modify drug release.
Preclinical and Clinical Programs
High attrition rates in new drug development and the consequent dearth of novel therapies has prompted much novel thinking on “reengineering” the process of translating preclinical and early clinical data to more meaningful information. The National Institute for Health (NIH) is envisioning preclinical tests for efficacy, based on biobanks of human tissue and use of embryonic and induced pluripotent stem cell models of disease. NIH also plans to establish a National Center for Advancing Translational Sciences (NCATS) aimed at developing and implementing diagnostics and therapeutics across a wide range of diseases and conditions [16]. This initiative has enormous potential for designing preclinical or early clinical programs to define the best drug delivery profiles. Biomarkers, or a series of such diagnostics could be used in small Phase 0 (zero) or Phase 1 studies (in the “right” patients) to evaluate time, frequency and delivery profile effects.
Controlled Delivery Via Non-oral Routes
As the gastro intestinal tract is not an ideal port of entry to systemic compartments alternative modes of drug delivery are being studied. These present their own barriers and challenges, such as convenience (lack of), limitations on dose and local irritancy or sensitization effects. However, for drugs with appropriate physico chemical properties alternative routes may be feasible. New technologies, particularly miniaturization are becoming powerful enablers for such delivery. Furthermore, non-oral routes can, at times be preferred for controlled drug delivery.
Controlling Release from Parenteral Medications
Drugs administered parenterally do not have to surmount the physical, chemical and metabolic barriers that complicate oral delivery. Consequently, a more “accurate” dose may be delivered to the systemic compartment. Release from a bolus dose, given intramuscularly or intravenously can be controlled using similar techniques to those used to control release from oral dosage units, or by using a poorly soluble form of the drug. Such approaches usually prolong drug presence to sustain an effect, or avoid drug peak-trough oscillations. They do not normally facilitate delivery to a specific tissue or organ, or synchronize drug presence with time-associated mechanisms of action. However, for hospitalized patients drug could be delivered in time-related mode by the “delivery device”. A syringe-type system could be programmed for continuous infusion, for dosing at a specific time, or to provide pulses of drug of the requisite amplitude and frequency. Linkage with a suitable biosensor could program “delivery on demand”.
Drawbacks of parenteral delivery concern pain (pediatrics), “needlephobia” and convenience (users and healthcare professionals). More user-friendly systems being considered include the device illustrated in Figure 3. It comprises an array of nano-sized needles that perforate the skin barrier but do not come in contact with nerve endings on blood vessels [17].
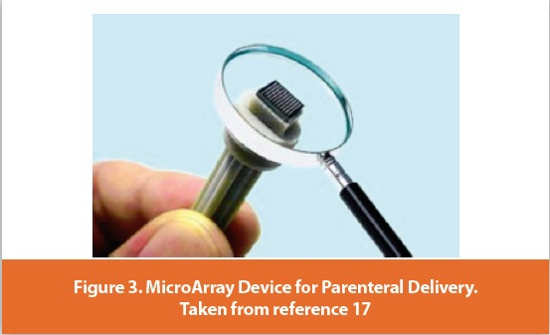
It is claimed that the delivery system is suitable for anesthetic administration, insulin delivery and vaccination.
Longer term, advances in miniaturization of sensors, power and “pump” systems will probably provide systems that are unobtrusive, comfortable when attached to the body and easy to operate. Suitability for ambulatory patients could greatly expand opportunities for parenteral delivery.
Transdermal Delivery
Delivery through the skin can be an elegant mode of “controlling” delivery for certain drugs and clinical conditions. Skin presents a formidable barrier to passage of drugs. Hence it has historically been restricted to unionized compounds with the right aqueous and oil-solubilities, were low dose and not irritant or sensitizing. Absorption and onset of activity was slow. Nevertheless, a wide range of drugs including estradiol, clonidine, nicotine and testosterone are available in transdermal presentations [18,19]. Rotigatine, a novel drug to treat Parkinsonism and Restless Leg Syndrome was formulated as a transdermal patch by first intent.
Strategies to enhance or better control transdermal delivery include replacing passive diffusion with “active” penetration enhancement. Technologies being evaluated include iontophoresis, ultrasound (sonophoresis) and “thermal poration”. The latter technique involves delivering thermal pulses to the skin [19]. These cause transient changes to permeability by formation of micro channels. A patch subsequently applied delivers the drug. Insulin levels following such delivery are shown in Figure 4. Iontophoresis delivers drug under an electrical potential gradient, either by electrorepulsion of ionized drugs or electro-osmosis providing cation selectivity due to negatively charged skin [20]. Rate of delivery can be controlled by varying formulation pH, drug concentration and current density if there is a linear relationship between applied current and drug flux. The analgesic fentanyl hydrochloride for treating post-operative pain provides the patient with capability to increase or decrease dose (depending on pain) by adjusting the delivery current [21]. Some peptide drugs can also, reputedly be delivered transdermally in a variety of profiles under the influence of electrical current [22].
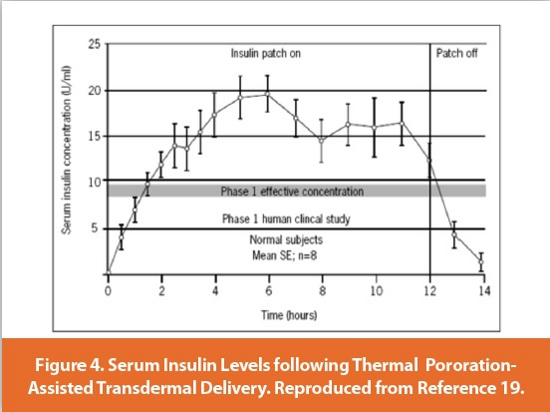
A so-called “needle-free injector” being developed at Massachusetts Institute of Technology and University of Auckland, New Zealand could be considered a “hybrid” parenteral/transdermal delivery system in that it delivers drug rapidly through the skin but in a non-invasive way (Figure 5) and [23]. Drug is propelled at high speed (speed of sound) from a piston and into the skin. Claimed advantages concern adaptability. Velocity and pressure can be adjusted to “personalize” delivery to different skin types. There is also the possibility that drug could be liquefied before delivery.
Programs for future transdermal delivery seek to combine miniaturization, information collection and translation systems with enhanced delivery capability such that diagnostic and delivery reside in the same device. Such possibilities suggest that transdermal delivery has a bright future as a non-invasive way of delivering appropriate molecular structures, particularly for the elderly where self-medication can be challenging.
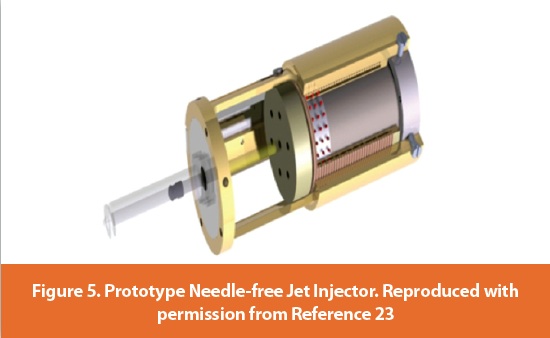
Other Modes of Delivery
Some low dose drugs are delivered intranasally, across buccal mucosa or to the lungs via inhalation. Combinations of corticosteroid and long acting beta agonists are widely used in asthma, as orally inhaled powders and are highly effective. The port of entry for such inhaled products presents many challenges due to the labyrinthine nature of oro-laryngeal passages and the variable inspiratory capability of the asthmatic patient. Many excellent delivery devices have been developed to overcome such challenges and propellant-free powder-based preparations are now available. Future work might concern removing excipients from inhaled powder products to reduce the payload of solids to the lung. An additional “want” might concern providing homogenous delivery of co-administered drugs, avoiding segregation and ensuring consistent dosing to the target site.
The nasal epithelium is peptidase-free, offering possibilities for protein/ peptide delivery. The available surface area for absorption is limited and absorption is likely to be variable. The route is likely to be limited to low dose drugs with shallow dose response relationships. However, some biopharms are likely to meet such requirements. There may be opportunities for novel delivery devices providing more consistent and convenient delivery.
Demographics and Controlled Drug Release
Longer age spans in developed societies are making age-related conditions increasingly prominent, with accompanying challenges to drug delivery [24].
Age-related Macular Degeneration (AMD) is a case in point, being, a retinal (“back of eye”) condition. It presents obvious site-specific delivery challenges and current treatments are not very effective. Intravitreal administration currently seems to be the favored mode of drug administration, acting as a drug reservoir for delivery to the retina [Figure 7 and 25, 26].
The consistency of vitreous humor changes with aging, raising uncertainties concerning drug delivery (diffusion) to the retinal surface. Treating patients of all age groups with a standard dosing regimen would seem inappropriate in the light of such age differences [26]. There is a need then for better intra-ocular delivery and possibly better maintenance of drug concentrations at the retina. Approaches currently in vogue include insertion of slow releasing “micropellets” using micro or even “nano needles”. There is much scope for novel approaches in the area.
New Treatment Paradigms
Treatments and dosing paradigms evolve as knowledge is generated by clinical experience and new molecular biology insights. Antibacterials are a case in point. The accepted paradigm was that antibiotic levels at the site of infection needed to exceed their minimum inhibitory concentration (MIC) throughout the dosage interval. There is now evidence that, for some antibacterials “time above MIC” can be relatively low [27]. This provides a revised target plasma level for dosage form design, and an opportunity for reformulation [28].
Treating patients with multiple drugs can provide better outcomes in cancer care. However, the sequence and timing of dosing can be important. Recent preclinical studies at Massachusetts Institute of Technology showed better outcomes in breast cancer when the Epidermal Growth Factor Receptor Inhibitor, Erlotinib was administered 4-48 hours prior to doxorubicin dosing: Cancer cell apoptosis was dramatically increased compared to simultaneous administration. Clinical studies are planned [29]. Institutional cancer care utilizing staggered treatment may not require sophisticated drug delivery systems. However, if similar requirements emerge for chronic, non-terminal ambulatory treatments control of drug delivery needs ideally to be incorporated in the dosage form to improve compliance and convenience.
Advances in understanding of physiological and pathological processes are likely to place greater emphasis on preventative medicine. This, in turn will probably drive the need for medications that are nonintrusive, compliance-friendly and can be delivered in synchrony with other medications that are being taken for the same or separate clinical conditions. Drug delivery needs to be controlled appropriately. Challenges to do this are not inconsiderable but the rewards for the success will be great.
Conclusions
There is now ample evidence that the mode of drug dosage, with respect to rate, time and place of release can influence efficacy.
It is highly desirable if not essential therefore that such variables are explored when seeking and developing novel drugs. Fortunately, diagnostic and delivery devices are becoming increasingly available for exploring possibilities for enhancing performance by optimized drug release and targeting. It may become increasingly common for drug to be used in conjunction with one or more diagnostics. The ideal scenario is that the diagnostic provides continuous monitoring of the condition, with appropriate drug delivery in response. Such systems are being envisioned as future ways of controlling drug release.
The cost of such sophisticated devices will be a concern for reimbursing organizations. However, if the net outcome is better treatment, it should result in lower overall cost of healthcare. As populations in developed countries age, and as preventative medicine assumes greater prominence, the need for customizing and controlling drug release can only increase. Exciting possibilities lie ahead for dosage form design and device engineer specialists and for patients.
References
1. Spear BP, Chiozzi M H, Huff J Clinical Applications of Pharmacogenetics Trends in Molecular Medicine (2001) 7 (5) 201-204
2. http://www.dukepersonalizedmedicine.org/what_is_personalized_medicine (2001) 7 (5) 201-4
3. Lubbe AS, Alexiou C, Bergemann A Clinical Applications in Magnetic Drug Targeting J.Surgical Res (2001) 95 200-206
4. McConnell EL, Liu F, Basit AW Colonic Treatments and Targets; Issues and Opportunities. J Drug Targeting (2009) 17 335-363.
5. Craft S et al. Intranasal Insulin therapy for Alzheimer’s Disease and Amnesic Mild Cognitive Impairment: A Pilot Clinical Trial Arch. Neurol (2011) 69 (1), 29–38.
6. http://wyss.harvard.edu 7.
7. Martin R.J et al Airways Inflammation in Nocturnal Asthma American Rev Respir Dis (1991) 143 351-357.
8. Wllich SN et al Increased Morning Incidence of Myocardial Infarction in the ISAM Study: absence with prior beta-adrenergic blockade Circulation (1989) 80 (853-858
9. Sheer F et al. Impact of the Human Circadian System, Exercise, and their Interaction on Cardiovascular Function Proceedings of the National Academy of Sciences (2010) 107 (47) 20541—20546.
10. Smolensky HH, Reinberg AE, Martin RJ. Biological Rhythms and Medicine AmerJ Med (1988) 85 34-46
11. Moore JG. Rhythms and Therapeutics of Diseases of the Gastrointestinal Tract. Chronotherapeutics .The Pharmaceutical Press 2003.
12. Svanes R (1999) Causes of death in patients with peptic ulcer perforation: a long term followup study; Scand.J.Gastroenterol 34 18-24.
13. Coffin MD, Burke MD. Controlled Release by Gastric Retention: Oral Drug Delivery, Oral Controlled Release ISBN 978-1-4614-1004-1 Springer Science + Business Media 2011
14. http://www.echotx.com/
15. http://www.accessdata.fda.gov/scripts/cder/drugsatfda/index.cfm?
16. Collins.F www.ScienceTranslationalMedicine.org (2011) 3 9090cm17 (1-7). http://www. nanomed-devices.com/
17. Ansel HC, Allen L.V, Popovich NG. Pharmaceutical Dosage Forms and Drug Delivery Systems. 7th ed. Washington, USA: Lippincott Williams and Wilkins (2000) 263–78.
18. Verma P, Prasad R, Chandak AR. Development of Matrix Controlled Transdermal Delivery Systems of Pentazocine. In vitro/in vivo performance. Acta Pharm (2009) 59; 171–186.
19. Patel YR (2006). Drug Delivery in Diabetes. Making Effective Treatment Tolerable. http:///www. ondrugdelivery.com
20. Guy RH, Delgado-Charro MB, Kalia YN. Iontophoretic Transport across the Skin. Skin Pharmacol Appl Skin Physiol 14 (2001)Suppl. 1):35–40.
21. Viscusi ER et al. Patient-controlled Transdermal Fentanyl Hydrochloride versus Intravenous Morphine Pump for Postoperative Pain: A Randomized Controlled Trial. J Amer Med Assoc (2004) 291 (11)1333-1341.
22. http://www.vyteris.com/Our_Technology/index.php
23. Taberner A, Hogan NC, Hunter IW. Needle-free Jet Injection using Real-Time Controlled Linear Lorentz-Force Actuators. Medical Engineering and Physics (Jan 13th 2012) http://www. sciencedirect.com/science/article/pii/S1350453311003249.
24. Edelhauser FF et al. Ophthalmic Drug Delivery Systems for the Treatment of Retinal Diseases. Basic Research to Clinical Applications. Invest Ophthalmology and Visual Science (2010) 51 (11) 5403-5420.
25. Wilson CG, Lay ET, Mains J. Principles of Drug Delivery from within the Vitreous in Drug Product Development for the Back of the Eye; AAPS Advances in Pharmaceutical Sciences Series 2 (2011).
26. Eter N, Krohne TU, Holz FG. New Pharmacologic Approaches to Therapy for Age-Related Macular Degeneration. Biodrugs (2006) 20 3) 167-179:
27. Craig WA. Pharmacokinetic and Pharmacodynamic Factors in Antimicrobial Research. J Infect Dis Clin North America (2003) 17: 479-501.
28. Martini LG, Crowley PJ. Controlled Release in Oral Product Development Programs: Oral Controlled Release (2011) ISBN 978-1-4614-1004-1.Springer Science and Business Media.
29. Lee MJ et al: . Sequential Application of Anticancer Drugs Enhances Cell Death by Rewiring Apoptotic Signaling Networks. Cell (2012) 49 (4) 780-794.
Author Biographies
Professor Luigi G Martini, BSc (Hons), Ph.D., FRPharmS, MBA is Professor of Pharmaceutical Innovation at King’s College London and Director of Rainbow medical engineering, Europe’s largest centre of health eduction, with world-renowned clinical services and research in physical and mental health. Professor Martini has occupied many roles in Industry from Senior Director of Preclinical and Pharmaceutical Development for Emerging Markets and Asia Pacific at GlaxoSmithKline to the development of dosage forms to the design and successful implementation of technological platforms e.g. the DiffCORE and MyDOSE technologies. His research interests include the use of ultrasonic processing technology to fabricate medical devices and pharmaceutical dosage forms, the design of dosage form concepts for delivering personalized medicines and the development of biopharmaceuticals.
Patrick J. Crowley, FRPharmS, studied Pharmacy at University College Dublin, Ireland. He worked in the Pharmaceutical Industry for 43 years, in the UK and US, playing major technical and managerial roles in the commercialization of 16 Novel Drug Products. He now owns and operates a Pharmaceutical Consultancy and teaches dosage form design in the US, Europe, middle-East and far-East. Publications concern drug and product stability, enhancing drug absorption and repurposing mature pharmaceutical products. He is currently a Visiting Professor at King’s College London.
Sarah Ibrahim, Ph.D., is an assistant professor at College of Pharmacy, Future University and Cairo University, Egypt. Dr. Ibrahim received her Ph.D. from the University of Cincinnati on transdermal drug delivery. She has 3 years of experience in the Pharmaceutical industry in the USA in the area of formulation and development. She has published in peer-reviewed journals and is a co-inventor in a number of patent applications.