Abstract
Displacement chromatography (DC) can be advantageous in comparison to gradient chromatography. Parameters favoring DC allow significantly larger sample loads since the binding capacity can be exploited more efficiently. As a result, columns that are up to ten times smaller are necessary for a given sample amount. Under appropriate conditions, the main product forms a broad band with high purity. A drawback of DC sometimes is the displacer, which is needed for eluting the product from the column. This problem can be circumvented by sample displacement chromatography (SDC). SDC requires that the sample molecules bind to the column in relationship to their affinities, thereby separating themselves. After sample loading on column segments connected in series is finished, the column is fragmented into segments and the separated products are eluted from the individual segments by a step gradient. This article demonstrates the simplicity and effectiveness of SDC for the separation of proteins.
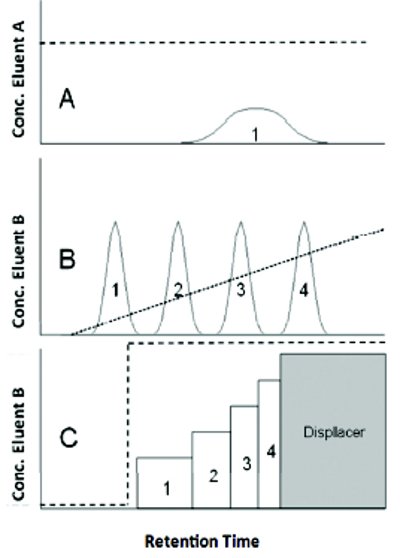
Figure 1. Scheme of the three basic modes of liquid chromatography: a) isocratic mode; b) gradient mode; c) displacement mode. Dashed lines represent time course of the relative eluent concentration. The solid line represents the time course of the relative concentration of the molecules 1-4, eluting from the column. In this idealized example, it is assumed that all four diff erent molecules are present in equal concentrations. The affinity of the molecules follows the order 1 < 2 < 3 < 4. It is assumed that the molecules 2-4 did not elute from the column under the isocratic mode (a) because of their higher affinity towards the stationary phase. Note, that under the mode displacement (c) molecules elute in bands (rectangular peak shape) from the column.
Introduction
More efficient chromatographic methods is an increasing need for purified proteins in basic life science research as in the pharmaceutical industry. In all cases, target proteins must be separated from components of the host cell system or from other sources, like tissue. The well-established gradient chromatography is associated with the disadvantage that the binding capacity of the column cannot be fully explored when a high resolution is desired. Displacement chromatography offers an alternative here, as up to 80% of the binding capacity of a column can be utilized [1]. Displacement chromatography (DC) is, along with isocratic and gradient chromatography, one of three basic modes of liquid chromatography [2] (Figure 1)). With DC, the sample is diluted in a sample application buffer, termed carrier, which promotes a high affinity of the target molecules to bind to the stationary phase of the chromatographic material. During sample application, the sample molecules will distribute themselves along the column according to their affinity to the stationary phase. As a result, the molecules with the highest affinity will be located at the top of the column and the molecules with lower affinity will be bound further away from the top of the column. Pumping continuously, a displacer containing eluent through the column will elute the adsorbed sample molecules. This step starts directly after sample application is finished. The displacer molecule, which should have a higher affinity towards the stationary phase than any other component of the sample, affects the displacement of the sample molecules as it passes down the column, thereby causing the sample molecules to arrange themselves in sample bands (displacement train) and elute in order of increasing affinities towards the stationary phase. The DC completes when the displacer totally saturates the stationary phase and thus elutes [3]. DC is beneficial for the preparative purifi cation of products from chemical synthesis [4] but can also be applied for the separation of molecules in complex mixtures [5], therefore used for proteomics [6-8]. Recently, DC has been utilized for the separation of protein species, which is one of the most challenging issues in protein separation and a big problem in the production of therapeutic proteins. (According to Jungblut et al. [9] Protein species are defined as proteins, which are coded by a single unique gene but differ in their exact chemical composition.) During the production of therapeutic proteins, different species of the same protein are generated. These species are often nonfunctional or even harmful when administrated to patients [10]. Zhang et al. demonstrated that a separation of charge variants of a defined mAb is possible with DC [11].
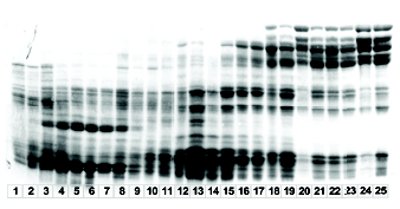
Figure 2. SDS-PAGE analysis of the eluates of an SDBC of a protein extract from renal tissue. The numbers represent the numbers of the chromatographic material segments. Tube number 1 was initially incubated with the sample. The last tube (number 25) contained the proteins with the lowest affinity.
Sample Displacement Chromatography (SDC)
DC requires the careful selection of an appropriate displacer [12] and the displacer may co-elute with the target protein thus making an additional separation step necessary. Sample displacement chromatography (SDC) circumvents the displacer problem, because SDC does not need a displacer. When separating a mixture of molecules with SDC, the dissolved sample is pumped onto several columns connected in series. During sample loading, the molecules compete for the binding sites at the stationary phase. Molecules with the highest affinity bind to the first column, whereas molecules with lower affinities bind to the subsequent columns. After sample loading has been finished, the columns are disconnected and the adsorbed molecules are eluted from each individual column segment [13]. With SDC, complex mixtures of proteins have already been separated [14].
Sample Displacement batch Chromatography (SDBC)
A drawback in SDC is that the binding capacity of the stationary phase in relationship to the sample must be determined because the sample size and the total binding capacity of the columns connected in series should be harmonized. If the sample size in relation to the total binding capacity is too small, this will result in an insufficient separation. In this case, most of the molecules will bind to the fi rst column. If the sample size in relation to the total binding capacity is too large, a part of the sample will not be separated [15]. Furthermore, the available column size may not be optimal in relation to the sample amount. For sample displacement chromatography performed in the batch mode (SDBC), this problem is less critical. Here the chromatographic material is handled in a buffer suspension allowing fl exible segment sizes. In addition, with the batch approach, even the chromatography of crude extracts is possible, which cannot be processed with columns due to the risk of column blocking. For SDBC, the total binding capacity is roughly estimated or experimentally determined yielding the total amount of chromatographic material, required for SDBC. An example for a typical calculation of the required amount of chromatographic material for SDBC is demonstrated in Kotasinska et al. [16]. After equilibration of the calculated total amount of chromatographic material in a sample application buffer, the suspension is distributed to reaction vials or tubes. After sedimentation of the chromatographic material, the supernatant is removed. The sample is applied to the first tube, incubated while continuously rotating the tube for keeping the sample, buffer, and chromatographic material in suspension. After settling down the chromatographic material, the supernatant is moved to the second tube. After incubation, the supernatant of the second tube is transferred to the third tube. Sample loading will be fi nished when the sample has passed the last tube. The chromatographic materials in the individual tubes are washed and thereafter incubated individually with an elution buffer for desorption of the bound molecules. In Figure 2, an SDBC separation result of a complex mixture of a protein extract from renal tissue is shown. The distribution of the pattern of protein bands demonstrates the high quality of the SDBC separation. A detailed description about the performance of SDBC is provided in Kotasinska et al. [16]. In this protocol, it is demonstrated that even a complex protein sample can be separated with SDBC. Furthermore, scaling-up an SDBC separation is shown to be very simple. All parameters simply must be multiplied with the up-scaling factor. Additionally, SDBC is a very inexpensive approach for the separation of proteins, which does not require sophisticated HPLC equipment. In order to achieve optimal results with SDBC, a screening of parameters is recommended, as described in Kotasinska et al. (15).
Conclusion
Sample displacement batch chromatography is a simple and inexpensive method for the purification of proteins. SDC as well as SDBC have no need for a displacer molecule still offering similar advantages and separation quality as DC. SDBC can be performed with a minimum consumption of chromatographic material. Easy up-scaling of parameters, determined by previous screening, makes SDBC well-suited for protein purification. The possibility of separating protein species makes this chromatographic mode also advantageous for large-scale separations in down-stream processing.
Author biography
Dr. Hartmut Schlüter is currently Professor at University Medical Center Hamburg Eppendorf. Professional affiliations include: American Society of Mass Spectrometry (ASMS), German Society of Chemistry (GDCh), German Society of Mass Spectrometry (DGMS), German Society of Biochemistry and Molecular Biology (GBM), German Society for Proteome Research (DGPF). He earned his doctorate from the Institute of Biochemistry, University of Münster. Dr. Schlüter can be reached via phone: +49 -40- 7410 58795 or e-mail: [email protected]
References
- H. Schlüter, J. Jankowski, in Protein Liquid Chromatography, M. Kastner, Ed. (Elsevier, 1999), chap. 11, pp. 505-524.
- J. Frenz, P. van der Schrieck, C. Horvath, Investigation of operating parameters in highperformance displacement chromatography. Journal of chromatography 330, 1-17 (1985);
- C. P. McAtee, Displacement chromatography of proteins. Current protocols in protein science / editorial board, John E. Coligan ... [et al.] Chapter 8, Unit 8 9 (2010).
- J. Jankowski, W. Potthoff , W. Zidek, H. Schlüter, Purification of chemically synthesised dinucleoside(5’,5’) polyphosphates by displacement chromatography. Journal of chromatography. B, Biomedical sciences and applications 719, 63-70 (1998).
- H. Schlüter, E. Offers, G. Bruggemann, M. van der Giet, M. Tepel, E. Nordhoff , M. Karas, C. Spieker, H. Witzel, W. Zidek, Diadenosine phosphates and the physiological control of blood pressure. Nature 367, 186-188 (1994).
- R. Ahrends, B. Lichtner, A. Bertsch, O. Kohlbacher, D. Hildebrand, M. Trusch, H. Schlüter, Application of displacement chromatography for the proteome analysis of a human plasma protein fraction. Journal of chromatography. A 1217, 3321-3329 (2010).
- M. Trusch, A. Bohlick, D. Hildebrand, B. Lichtner, A. Bertsch, O. Kohlbacher, S. Bachmann, H. Schlüter, Application of displacement chromatography for the analysis of a lipid raft proteome. Journal of chromatography. B, Analytical technologies in the biomedical and life sciences 878, 309-314 (2010).
- M. Trusch, K. Tillack, M. Kwiatkowski, A. Bertsch, R. Ahrends, O. Kohlbacher, R. Martin, M. Sospedra, H. Schlüter, Displacement chromatography as first separating step in online twodimensional liquid chromatography coupled to mass spectrometry analysis of a complex protein sample--the proteome of neutrophils. Journal of chromatography. A 1232, 288-294 (2012).
- P. R. Jungblut, H. G. Holzhutter, R. Apweiler, H. Schlüter, The speciation of the proteome. Chemistry Central journal 2, 16 (2008) 10.1186/1752-153X-2-16.
- V. Richter, M. Kwiatkowski, M. Omidi, A. Omidi, W. D. Robertson, H. Schlüter, Mass spectrometric analysis of protein species of biologics. Pharm. Bioprocess. 1, 1-24 (2013).
- T. Zhang, J. Bourret, T. Cano, Isolation and characterization of therapeutic antibody charge variants using cation exchange displacement chromatography. Journal of chromatography. A 1218, 5079-5086 (2011).
- K. Rege, A. Ladiwala, S. M. Cramer, Multidimensional high-throughput screening of displacers. Analytical chemistry 77, 6818-6827 (2005).
- R. S. Hodges, T. W. Burke, C. T. Mant, Multi-column preparative reversed-phase sample displacement chromatography of peptides. Journal of chromatography 548, 267-280 (1991)
- M. Brgles, J. Clifton, R. Walsh, F. Huang, M. Rucevic, L. Cao, D. Hixson, E. Muller, D. Josic, Selectivity of monolithic supports under overloading conditions and their use for separation of human plasma and isolation of low abundance proteins. Journal of chromatography. A 1218, 2389- 2395 (2011).
- M. Kotasinska, V. Richter, J. Thiemann, H. Schlüter, Cation exchange displacement batch chromatography of proteins guided by screening of protein purification parameters. Journal of separation science 35, 3170-3176 (2012).
- M. Kotasinska, V. Richter, M. Kwiatkowski, H. Schlüter, in Protein Downstream Processing, N. Labrou, Ed. (Springer, 2014), vol. 1129, chap. 24.