Abstract
Supercritical Fluid Chromatography (SFC) expedites both chiral and achiral separations by enabling analysts to resolve the more challenging sample mixtures rapidly with less solvent usage than with conventional HPLC. SFC methods development can be time consuming as small changes in method parameters such as modifier strength, column type, pressure and flow rate conditions can greatly impact the separation. In addition, the desired resolution can be unpredictable and challenging to obtain for chiral and achiral separations. For preparative scale separation, even higher resolution is often required for high sample load capacity. Therefore, investigating column and mobile phase selectivity is a necessary and crucial step to achieve resolution in SFC method development. Screening multiple columns simultaneously could significantly increase method development efficiency. This study introduces a novel, flexible parallel/multi-column setup for method development screening that is four times faster than traditional single column methods development.
Introduction
In today’s pharmaceutical discovery/development environment, companies encourage a “do more with less” approach to be more competitive and cost effective through adaptation and innovation. High throughput method development screens are successfully adapted to determine analytical scale enantiomer purity with methods such as CE, GC, HPLC and SFC [1]. Though high throughput approaches are the most efficient at separating and identifying enantiomers on this small scale; preparative chromatography has been the most widely used technique for preparing enantiomers in drug discovery. Currently, most chiral HPLC separations use normal phase to readily dissolve and separate samples using heptane or hexane with a polar organic modifier like ethanol or isopropanol. Normal phase HPLC multi-column-parallel screening approaches expedite this chiral method development for semi-prep and pre- scale needs [4].
Semi-preparative and preparative SFC methods are increasing in popularity as they further reduce costs by providing enantiomers or diastereomers with less solvent consumption as compared with HPLC preparative methods [1]. Once an appropriate SFC chiral method is found; an analyst can quickly provide the chemist with the appropriate amount of chiral material (50-100 mg each enantiomer) using semi-preparative SFC. Obtaining pure chiral material on this scale first, provides individual enantiomers both for fast initial biological testing and for chiral reference standard for future enantio-selective synthesis. Therefore, better scalable semi-preparative chiral methods can effectively result from screening as many different types of stationary phase and mobile phase combinations, in the shortest time possible.
Single-column isocratic screens are time consuming and wasteful. The stepwise isocratic screen takes a long time to identify the initial-chiral recognition conditions. In addition, an isocratic condition may not elute the compounds being analyzed within a reasonable time. [9]. Therefore, the screen method can be enhanced by adding a modifier gradient to encompass a wider range of compounds. Analytical gradients are also effectively scaled to preparative separations with mass directed SFC [10].
SFC parallel approaches currently use between four to eight columns on one system [5-8]. These multi-column screening approaches allow the user to rapidly identify the right combination of mobile phase and column to achieve the desired separation. These systems are limited to the one bank of eight columns selected. The multiple-column approach described here allows an analyst to switch among banks of four columns to have as many as sixteen column combinations. We achieved this by using readily available instrument components (detectors and switching valves), without a major capital purchase.
SFC is often perceived as having undesirable limitations compared to Reverse Phase HPLC [11]. These limitations can include an inferior signal-to-noise, especially at the higher percentage of co-solvents required to elute more polar analytes. Although an increasing body of knowledge demonstrates that SFC has improved peak resolution, selectivity and column efficiency compared to RP HPLC, the limitations have prevented a more complete adoption of SFC as an alternative to Reverse Phase HPLC, particularly in pharmaceutical development.
However, as SFC is increasingly adopted, one way researchers have found to overcome some of these limitations is through the use of water as a mobile phase additive. The addition of water has helped extend the range of polar compounds that can be resolved by SFC, as well as improved peak shape and resolution of especially polar molecules [12]. For example, the chromatography of fatty acids was shown to improve in pure CO2 capillary SFC with the addition of 1% water to increase the polar strength of the modifier [13]. Underivatized carbohydrates and mono- or polysaccharides were separated efficiently with the use of achiral SFC with the addition of between 0.2% [14] to as much as 8% water [15] and methanol with or without triethyl amine on achiral SFC columns. Formic acid or ammonium acetate with water as a modifier additive have expanded the scope of SFC/MS to complement reverse phase HPLC. SFC with MUX-MS with changes to both hardware and software analysis capability have kept pace with the increased demand from combinatorial chemistry [16].
Reducing the time needed for initial method development will free time, for example, to optimize the prep loading potential to maximize the injection stacking efficiency [17]. The method scouting time can better be used to optimize methods for future preparative scaling.
The SFC approach described here employs multiple 4-column parallel screens with 10-50% co-solvent scouting gradient methods, to increase the number of different column choices available for simultaneous chiral or achiral screening. This strategy can be implemented on most analytical systems to provide scalable multiple-column SFC configuration using readily available variable wavelength detectors and manual switching valves.
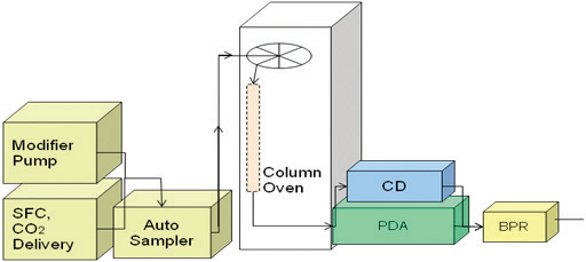
Figure 1A - A single-column system uses 2 or 3 mL/min flow with a temperature cooled fluidics system to convert CO2 to liquid in line with a photo diode array (PDA) and circular dichroism detector (CD). The back pressure regulator (BPR) connects through the CD to the PDA through a “T” connector to ensure the entire system is maintained at the required separation pressure (100-200 bar).
Traditional System of Single-Column with Multiple-Column Switching
A traditional SFC system provides a single column to be run at a time often with a switching valve to provide a number of column choices to be switched in-line. Figure 1A shows a one column, one run schematic configuration used for most traditional SFC systems. There is a modifier pump that can be switched to pump up to six different modifier solvent types separately through to a 6- or 10- position column switching valve. The switching valve can individually switch to these 6 or 10 column types, separately, one at a time per run. These systems are often equipped with a photodiode array and circular dichroism detector in- line for determining the spectra. Each separate individual method uses a specific modifier type and column type. Method development with these single-column systems is time consuming since each change for each column is done sequentially. Any system designed to do one-column-one method, can be converted to a parallel system with a 6 or 10-port solvent selector valve and additional UV detectors.
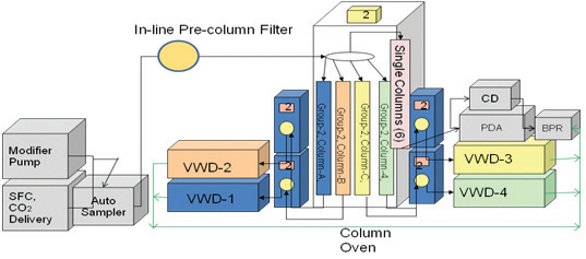
Figure 1B - The multiple-column SFC system adds four variable wavelength detectors (VWD1-4), through four 6-port selection valves directed to a given column group through a master control switch-shown in position 2.
A Novel Multiple Parallel-Column System Design and Setup
The four-column-at-once setup divides the system flow among four columns at once to quickly determine the separation efficiency of multiple columns for both chiral and achiral separations (Figure 1B). The current system is a retrofit of the 10-port selection valve system. Inside the oven are two 10-port solvent selection valves which are switched in unison by the software. A pulseless pump delivers 8 mL/min through the top 10-port selection valve inside the column oven. Each of the first four ports contains a bank of four columns which are plumbed through individual 6-port solvent selection valves in line with four dedicated Variable Wavelength Detectors. The 6-port selection valves are controlled by a central switching valve which allows the user to manually select the flow path (ports 1 through 4) for all four valves in unison, each corresponding to the same port on the 10-port oven valve. The user can then manually switch both the 10-port column valve and the 6-port column valve in unison to select a new bank of 4 columns in parallel. This configuration allows us to screen up to 16 columns in 4 injections. This also leaves 6 additional ports free in the column oven which are used for further single-column method optimization. The single columns are plumbed directly to the second (bottom) 10-port column selection valve inside the column oven, which directs the flow to a single photodiode array detector (PDA). The flow from the PDA is then passed through a chiral detector for collecting the CD signal. The backpressure of the entire system is controlled by redirecting the combined outlet from all the system detectors through a union prior to the backpressure regulator.
The setup is designed to provide as much information about an analyte in as short a time as possible. This research provides the analyst a more focused direction to further optimize the method beyond this initial screen.
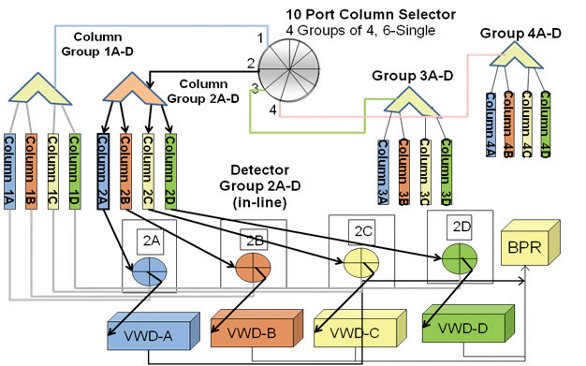
Figure 2 - A 10-port valve (in gray) attached to 6 single columns directly and 4 x 4-way splitters with a column attached to each. If column group 2, containing columns A to D is used, each detector goes to position-2 to indicate one column/one detector for the four columns in group-2.
The number of columns per group one can add is limited by the software’s capability to collect four analog detector signals at one time. Figure 2 provides a more detailed look inside the column oven where a 10-port valve includes connections to four parallel and six singlefunction columns. If the detector switching valve in Figure 2 is changed to position 1, for example, columns 1A to 1D of group 1 are then directed to the same four detectors but now through position three from each of the respective valves. This system at 4 columns per group has the addon potential for two more groups of four columns to the current 4 x 4 by removal of two of the six single columns to make 4 x 6. This would use all six positions of each detectors switching valve.
Hence, the column selection groups provide stationary phase selection four at-a-time to perform method screens four times faster than the traditional single-column approach. Furthermore, the six separate individual columns can still be used for single-column method optimization, semi-prep collection or post-sfc purity verification.
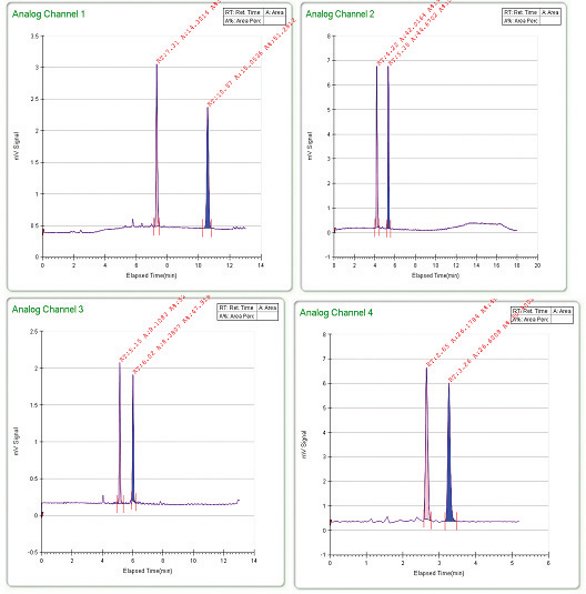
Figure 3 - System suitability test for four parallel chiral columns from one injection of trans-stilbene oxide (TSO) on parallel screening group 3 (3A to 3D) applying a gradient from 10% methanol, 90% CO2 to 50% methanol, 50% CO2 in 10 minutes, hold for 2 minutes and ramp back to down to 10% in 3 minutes. (the axes units are response in mV (absorbance at 220 nm) for y and retention time in minutes for x.)
System Setup Performance Testing
An appropriate chiral system performance test for each column per group assists in the adjustment of column pressure to ensure that columns in a given group are flow compatible. Trans-stilbene oxide was used here to determine column performance. A methanol modifier gradient was used to cover the range of separation polarities from 10% to 50% over 10 minutes, and a flow rate of 8 mL/min/4 columns or 2 mL/min/column (Figure 3) . A gradient from 10% to 50% over the parallel run also was used to provide a larger range of solvent modifier strength. Being able to vary modifier types on four columns at a time also enahnces the method development speed. Methanol was used as the primary screening modifier with gradient from 10% to 50% for these data.
The back pressure regulator will be sensitive to any leaks, so with the added multiple column complexity is the added difficulty of detecting leaks. It is recommended to always carefully monitor the pressure of the system throughout the run. The critical issues of solubility will not go away but only cause more problems in a system of this complexity.
Providing a pre-filter after the auto-sampler is critical to minimize potential clogging of some of the columns that will be difficult to locate and remove. Also, pressure balancing for each column in a given bank was done by the addition of varying length of small 0.005 mm restrictive tubing at the column outlet for appropriate pressure/flow balancing. A change of retention time is a key indicator of a shift in the balanced pressure/flow.
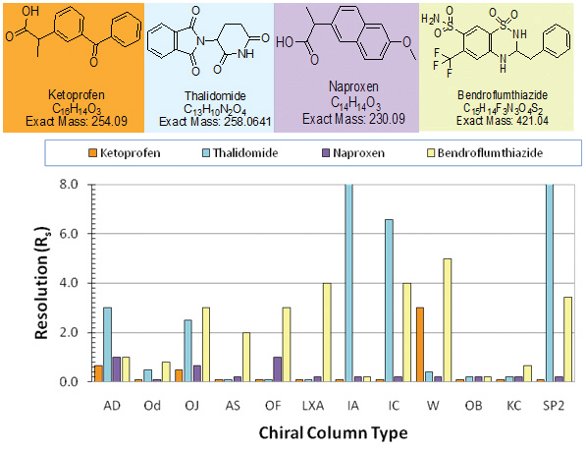
Figure 4 - Observed gradient resolution summary from multiple column parallel screening with just 3 injections each of four test compounds with chiral resolution (Rs=t2-t1/0.5 (w1+w2). At a glance, one can determine the chiral selectors for each for further optimization. (An arbitrary Rs of 0.2 was used as a placeholder for the bar graph when no resolution was observed).
Effectiveness of Multiple Column Chiral Screens
Chromatographers utilizing a multiple-column approach can determine, at a glance, which stationary phases separate enantiomers most effectively. Four commercially available chiral drug substances were used to demonstrate the performance of this parallel column approach. Ketoprofen, naproxen, thalidomide and bendrofluthiazide ran in three separate injections on three 4 x 4 chiral column groups. The graph in Figure 4 enables one to quickly identify which stationary phases offer potential resolution. This expedites screens for further method optimization.
Multiple-column screening as described here is one adaptation or step in the right direction to meet the flood of chiral and achiral separation requests, in an environment of increasing analytical and preparative expectation in discovery biopharma. These screens are also an advantage for diastereomers that may include structures with multiple “fixed” chiral centers and when solubility challenges limit modifier choices.
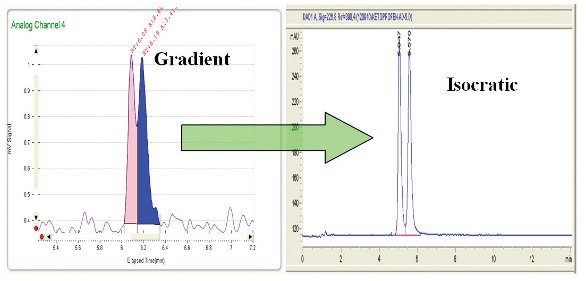
Figure 5 - Improved resolution of ketoprofen from the multiplecolumn gradient screen (Rs~1.3) to baseline resolution (Rs~2.2)
The clear advantage of multiple column screening is to fully utilize “all” the orthogonal information. One should pay attention to structural motifs, especially when acids or free base (primary amine) functional groups are present. There are cases where acid and base additives will be needed. Peak broadening may obscure chiral recognition, so the modifier may require other ternary additives such as diethylamine or formic acid. Figure 5 shows how the partial resolution of ketoprofen enantiomers on the AD column from the previous parallel screen information can be further optimized.
The initial AD column screen conditions were further adjusted on single-columns to obtain baseline resolution with just two additional injections beyond the initial screen. Isopropanol replaced methanol, and 0.1 v/v% formic acid was added to the isopropanol.
Multiple-column Parallel Approach for Effective Achiral Method Development
Multiple-column and parallel method enhancements also change the potential of SFC development for achiral analysis. Non-chiral products that require high purity are typically analyzed using reverse-phase purity methods.
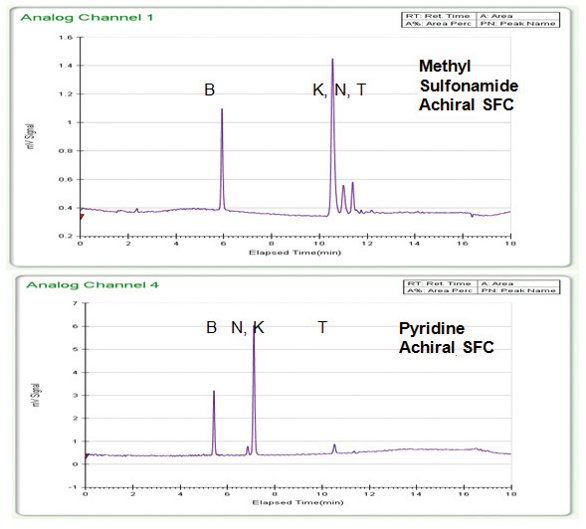
Figure 6 - Selected results from two of four parallel achiral SFC columns used with achiral group 4 that provided orthogonal selectivities as shown by the order of the performance test analytes top: Bendroflumthiazide (B), Ketoprofen(K), Naproxen (N) and Thalidomide (T); bottom, Bendroflumthiazide (B), Naproxen (N), Ketoprofen (K) and Thalidomide (T).
This multiple-column system screen also works well with non-chiral column types for example, 2-ethyl pyridine, amine, cyano, diol, silica, methyl sulfonamide to name a few, have very different stationary phase compositions and component elution order. Two of these examples, the methyl sulfonamide and pyridine column, (Figure 6), show completely different elution selectivity. These elution affinity differences can be a tremendous benefit when resolving impurities from the main analyte.
We have successfully developed SFC methods using achiral columns with equivalent resolution efficiency to reverse-phase HPLC. These SFC methods are typically applied to achiral material to provide pure reference standard using SFC.
Single-column to Multiple-column Screening Considerations
The Gradient Advantage
The one-column-at-a-time, trial and error is divided into four to streamline stationary phase changes. One can also select a different solvent type for these multiple-column parallel screens. These advantages, coupled with use of modifier gradients from 10% to 50% can rapidly identify both chiral and achiral separation potential and the analyte elution strength. Multiple-column SFC screening with gradient provides a much more practical means to develop methods for molecules with wide diverse structures and polarities. The CO2/modifier ratios range from 90/10 to 50/50 to provide an appropriate means to elute any analytes or highly retained components from non-polar to very polar. Once the chiral stationary phase and elution time/ modifier strength of the enantiomers are known, a traditional single-column development can be used to optimize the resolution.
The Need to Maintain Single-column Capability on Multiple-column Systems
Multiple-column screens work effectively when either a major structural change is made to a common core molecule or when an unfamiliar chiral motif requires entirely new chiral methods. The latter is most common in the discovery area, so screening in parallel is much more advantageous than traditional single-column method development. Any multi-column parallel system should, however, provide the ability to run single-column methods once a chiral stationary phase and solvent condition are identified. These single-column ports are critical for final method optimization and preparative final fraction purity checks.
Always Consider Prior Experience with a Chiral Molecular Series
Analytes with minor substitution differences or small functional group changes to the core may also successfully be separated with chiral methods that worked for similar motifs in the past. One should always seriously consider what successfully worked in the past, before running a complete chiral screen for structures with the same chiral core and minor functional group changes. In many cases, experiences with solubility, modifier type and sometimes even the chiral selector will prove to be important for the method development. Maintaining a single-column method optimization capability is, therefore,critical.
Preparative Considerations
The ultimate goal for method development screening is often to develop chiral or achiral methods that one can be scaled up for preparative resolution to obtain material of high purity. This screening approach rapidly provides a starting method that can then be optimized for both chiral and achiral prep. Although many methods provide some chiral recognition, the difference between a resolution (Rs) of ~1.3 and ~2.2 shown previously (Figure 5), for example, can translate to a reduction in hours or even days when scaled to preparative SFC separation.
We have been successful providing a number of very highly pure products with much less solvent evaportion by using non-chiral SFC prep methods. This multiple-column screening will be beneficial to constructing method separation libraries for multiple compounds. Identifying one condition that works for multiple analytes avoids costly preparative column changes and new modifier re-equilibrations when possible. Once data is collected in parallel it can be interpreted on a per condition basis, for further optimization to maximize sample loading or impurity resolution as needed.
Conclusion
Selecting the most promising column and mobile phase condition in the shortest amount of time allows chromatographers to focus on more definitive details for method optimization, purification and isolations for both chiral and achiral resolution. These simple modifications to existing equipment will reduce cost by maximizing the run-time efficiency. The results of different columns are now obtained four times faster than a one-injection, one-column condition. This four-column-at-once approach expedites both chiral and achiral SFC method development. These current improvements, coupled with this transition from single sample to multiple column gradients allow the analyst to meet the increasing demands of the biopharmaceutical industry within reasonable cost constraints.
Acknowledgements
The authors would like to thank David Wang Iverson for very helpful manuscript recommendations and organization and Adrienne Tymiak for the support and funding for these much needed implementations.
References
- Moran, P. Annales Pharm. Francaises (2009) 67, 241-250.
- Finn, M.G.In Chiral Analysis, Busch K.W.; Busch M.A. Ed. , 2006, Elsevier, Ch 4 pp. 79-93.
- Eliel E.L.; Wilen, S.N. Eds. In Stereochemistry of Organic Compounds, 1994, John Wiley & Sons, Ch7 Separation of Stereoisomers. Resolution. Racemixation, pp. 297-359.
- Zhang, Y.; Watts, W.; Nogle, L.; McConnell, O. J. Chromatogr. A, (2004) 1049, 75-84
- Subbarao, L.; Wang, Z.; Chen, R. “Introducing Parallel SFC System for Fast Method Dev. Optimization” unpublished draft manuscript with parallel SFC.
- Laskar, B.B. Chirality, 20 (8) (2008) 885-895.
- http://www.zinsserna.com/chiral_screening.html , web article entitled “Parallel Chiral Column Screening with SFC”; regarding the 8x Sepamatix © 2009.
- Zeng, L.; Laskar, D.B.; Kassel, D.B. J. Chromatogr. A. 1169 (1-2) (2007) 193-204.
- Desmet, K.;Sandra, P.;Vizvardi, K.; Hoornaert, G.J.; Van der Eycken, E. J. Microcolumn Separations, 13 (4) (2001) 163-168.
- McClain, R.; Dudkina, A.; Barrow, J.; Hartman, G.; Welch, C.J. J. of Liq. Chrom. And Rel. Tech., 32 (2009) 483-499.
- Wang, Z.; Liu, O.; Donovan, B. Am. Pharm. Rev. 2009, 10, 59-63.
- D.Moore,M. Hicks, A. Apedo, T. Gaskill, Y. Zhang “Waters as an SFC Modifier to Enhance Chiral Resolution of Polar Enantiomers”- Poster BMS BMSIARC 2010-unpublished results.
- Pyo, D. Lee, S. Bull. Korean Chem. Soc. 1999, 20, 4, 405-407.
- . Bolanỗs B. et al. Inter. J. of MS. 238 (2004) 85-97.
- Salvador, A.; Herbreteau, M.; Lafosse, M.; Dreux, M. J. Chromatogr. A. 785 (1997) 195-204.
- Farrell, W.P.; Arugemma, C.M.; Masters-Moore, D.F. J. Liq. Chromatogr. Relat. Technol. 2009, 32/11-12, 1689-1710.
- Stringham, R.W.; Krueger, B.R.; Marshall J. Chromatogr. A.1175 (2007) 112-116.
Author Biographies
Dr. Michael B. Hicks is a Senior Research Investigator I in the Separation Physical Sciences Group, Bioanalytical Discovery and Analytical Sciences at BMS Hopewell, NJ. He has 20 years of pharmaceutical experience with a diversified analytical research background ranging from early discovery to pharmaceutical pre-clinical R&D. He started in AR&D in 1989 at Merck & Co., Inc. Rahway, NJ. He received his Ph.D. from Seton Hall University in 1997. Mike previously worked in PK group at Merial. He led the Bioanalytical R&D Group at Polymerix Corporation, Piscataway in polymer drug delivery. On Saturdays in the Spring semesters, Mike leads the Advanced Biomedical Engineering Graduate Laboratory at Stevens Institute of Technology as an adjunct professor and serves on the Board of Directors for EAS. He has over 12 presentations and 10 first author publications in peer-reviewed journals.
Dr. Yingru Zhang is Principal Scientist at Bristol-MyerSquibb Company (BMS) leading the Separation and Physical Science groups in NJ for pre-clinical drug discovery and optimization. He obtained his Ph.D. in Physical Chemistry under Prof. Aronson at City University of New York in 1990. He was a Principal Research Scientist at Wyeth prior to joining BMS in 2004, and had worked in both Development and Discovery at American Cyanamid Co. that later became Wyeth (now Pfizer) for 12 years. He also established a multifunctional analytical department at Synthon Chiragenics Corp. Dr. Zhang had 16 publications in peer-reviewed journals, pioneered Simulated Moving Column (SMC) technique for chiral-HPLC and SFC, and developed Multi-Column Parallel Screening with Circular Dichroism Signal Pooling for rapid chiral method development in 2004.
Douglas Moore is a Research Scientist in the Separations and Physical Sciences Group, Bioanalytical and Discovery Analytical Sciences at Bristol Myers-Squibb in Hopewell, NJ, specializing in chiral separations method development and preparative resolution by HPLC and SFC. Doug has over 20 years of analytical chemistry and project management experience in the environmental, agricultural and pharmaceutical fields. Prior to joining BMS, Doug supervised a pesticide formulation testing lab and also analyzed agricultural products with the feed chromatography lab for the Office of Indiana State Chemist. Doug graduated in 1986 with a Bachelor Science degree in Chemistry from Guilford College in Greensboro, NC.
Atsu Apedo is currently pursuing his Ph.D. at Seton Hall University, expecting to graduate in Fall 2010. His research is focused on multidimensional analysis using, HPLC, GC and TOF. As Research Scientist in the Discovery Analytical Sciences, separation group at Bristol Myers Squibb Co he joined BMS in 2001, from Wyeth-Ayerst, Monmouth Junction, where he worked as research scientist in Medicinal Chemistry II. He has a BS in Pharmaceutical Science and a MS in Pharmacology from, China Pharmaceutical University.
This article was printed in the September/October 2011 issue of American Pharmaceutical Review - Volume 14, Issue 6. Copyright rests with the publisher. For more information about American Pharmaceutical Review and to read similar articles, visit www.americanpharmaceuticalreview.com and subscribe for free.