Abstract
Mixtures of unknown materials may be found during the development and manufacturing of biopharmaceutical drug products. Their physiochemical properties must be identified to determine the root cause and to take corrective and preventive actions. Fourier Transform Infrared (FTIR) spectroscopy is widely employed as a powerful analytical tool to obtain the fingerprints of unknown materials in the Mid-IR region. It has been widely applied to identify unknown material with the assistance of contemporary commercial IR libraries where a large number of reference IR spectra are available; however, identifying mixture of materials with only FTIR library searching is a challenge as most reference spectra in the IR library originate from pure compounds. In this work, microscopic-FTIR and limited spectral range searching technique along with the background information of the materials used in the manufacture processes were utilized to resolve and identify mixtures of unknown materials in biopharmaceutical manufacturing from two incident cases. The selected cases illustrate the importance of combining microspectroscopic techniques with spectral library searching, functional group analysis, and knowledge of the materials used in the processes for manufacture incident investigation.
Introduction
Proper control of raw materials, equipment, and containers during pharmaceutical production is of utmost importance because of the potential detrimental effect the contaminants can have on the well-being of patients [1]. The unknown contaminants manifested in the downstream processes occur more often than those of upstream processes. The identification of trace amounts in a mixture of materials is not an easy task. An example would be crime scene samples, which need special handling and are often in limited quantities [2]. GC (Gas Chromatography) [2] and HPLC (High Performance Liquid Chromatography) [4] are widely used to separate mixtures of materials for further identification by mass spectrometry or spectroscopy; however, the materials need to be vaporized or dissolved in solvents. Both techniques, which may require relatively large amounts of samples, can be sample-destructive and time consuming. FTIR requires only a small quantity of unknown materials and has the advantages of rapidity and simplicity [1]. Additionally, the FTIR microscopy is relatively nondestructive, although the compression cell may flatten the morphology of the samples [1]. Identifying unknown compounds by matching spectra of the unknowns with library spectra of known molecules is a common practice. Rapid developments in computer technology and FTIR instrumentation have resulted in many electronic FTIR libraries on the commercial market [5]. FTIR library searching has been successfully applied in many fields including automobile paint attribution [6], pharmaceutical impurities [7], disease diagnosis, [8] and prion search [9].
Some previous publications demonstrated the power of FTIR in identification of foreign materials in biopharmaceutical production [1] and classification of cartilage quality in human tissue [8]. However, the identifications in reference [1] paper handled single component unknowns or a mixture with similar functional groups (gluconate and gluconatic acid). The reference [8] paper developed a successful model to detect cartilage degradation, but without clearly identified the molecular structure. The identification of mixture of materials with very different functional groups presents a great challenge for library searching since most spectra in the library are pure compounds. Light microscopy coupled to the FTIR spectrometer was applied to measure the mixed cultures of microcolonies (50-100 μm) [10, 11]. By using this coupled microscope, a mixture of microcolonies was resolved, and each microcolony was thus identified. Some spectroscopic researchers have developed special algorithms to handle mixtures of compounds. The concept of searching mixtures in an FTIR library was first developed by Nyden et al [12]. Their algorithm treated unknown mixtures as a linear combination of the spectra in the library after transforming the library to an orthonormal set of spectra. The method worked well for a small library of 10 spectra, but it is unable to process larger libraries containing spectra of similar compounds. Modern FTIR libraries can have over 200,000 spectra in the database, and it is very challenging to search mixtures against such a giant database and get meaningful identification of the individual component. The role of spectroscopists is critical for the identification of these mixtures using functional group analysis and knowledge of the materials, the product, and the process to supplement library searching. The two case studies in the present paper illustrate how to approach mixtures of biopharmaceutically relevant unknown materials. Functional group analysis was applied to scrutinize the list of potential matches and make the final identification in all cases.
Materials and Methods
Materials
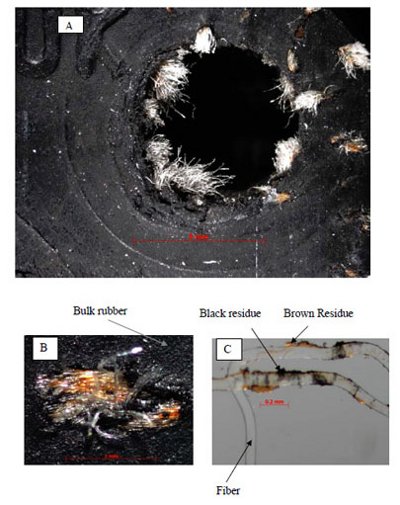
Figure 1 - (Case 1) A: Overview image of the piece of manufacturing gasket, B: Zoom-in of a bundle of fibers and C: the flattened fibers. There are four representative materials: bulk rubber, fiber, black residue on fibers and brown residue on fibers.
Gasket Sample (Case1): A piece of the gasket was submitted for a detailed analysis of the material composition of the gasket. The gasket is a black elastic composite material with fibers protruding from the hole of the gasket (Figure 1). The fibers were then pulled out from the gasket with black and brown residues on the fiber surface. The separated materials were then transferred onto NaCl plates and flattened by the compression cell for FTIR analysis. Four components were manually separated and identified with the assistance of library searching.
Particles in Formulated Drug (Case 2): Forensic analysis was requested to assist root cause investigation of the particulation phenomena in biopharmaceutical solution in vials. The solution with particles was filtered onto a gold coated filter. These filters have a pore size of 0.8 μm and were fixed on a circular aluminum frame with a diameter of 25 mm. With the reflective surface, the FTIR spectra of samples were collected without picking the micro-particles under a stereomicrocope and transferring onto special IR accessories, which is a very tedious and challenging task.
Methods
Optical Microscopy: All samples were initially examined with a stereomicroscope. The images of the particles were captured utilizing the color digital camera on the instrument and recorded on a computer connected with the optical microscope. In Case 1 the manipulations of micro-particles were performed under the microscope to assist in the visualization of the particles.
FTIR Microspectroscopy and Library Searching: The filter in Case 2 was loaded directly on the sample stage, and the FTIR spectra were obtained with the microscope in reflection mode. The micro-particles of Case 1 in compression cells (NaCl) were measured in transmission mode. The spectral range of collection was set from 4000 to 400 cm-1. Each FTIR spectrum was collected with 128 scans with 4 cm-1 spectral resolution. The spectra were recorded and processed (baseline corrected if needed) with FTIR instrument software prior to library searching. Library searching was performed. FTIR searching automatically examines hundreds of thousands spectra in the database and enables us to compare the unknown spectra with the most similar spectra. The algorithm is based on pattern matching and does not incorporate factors, such as the background information of the unknown materials, into the Hit Quality Index (HQI) ranking. A relatively high HQI of 900 is very helpful to condense the number of similar materials to a potential match; however, it alone cannot determine the identification. The library search may give wrong hits, or it may only be able to identify one component of a mixture. Under these situations, background information and spectroscopic knowledge are more important to obtain correct and complete identifications.
Results and Discussion
Identification of Unknown Materials in a Gasket in Fill and Finishing Facility (Case 1)
The gasket was found to have bundled fibers protruding out of the bulk rubber as shown in Figures 1A. When the bundle of fibers was analyzed under increased magnification, a brownish color could be observed in the areas close to the rubber matrix (Figure 1B). Some fibers were pulled out with micro-surgical tools, and a few residues were found around the fibers (Figure 1C). There were four representative materials found in the gasket: black bulk rubber, fibers, black residue, and brown residue. These representative materials could not be measured separately with a conventional FTIR spectrometer. Microscopic FTIR has the advantage of spatial resolution power, which allows one to focus on an area as small as 10 μm. We therefore could focus the beam on all representative materials and collect separate IR for each. The individual IR spectra can be searched separately.
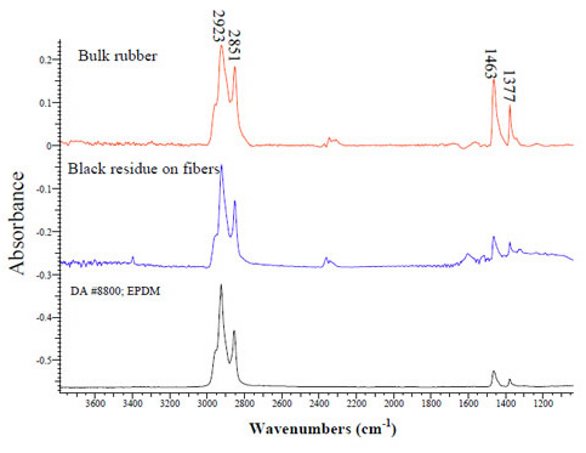
Figure 2 - (Case 1) FTIR spectra of the bulk rubber and the black residue on fibers. A library reference of EPDM (Ethylene Propylene Diene Monomer) is also shown. The bulk rubber and the black residue on fibers were both identified as EPDM.
The FTIR spectra of the black bulk rubber and the black residue were searched in the database, and they were both identified as EPDM (Ethylene Propylene Diene Monomer (M-class) rubber). “M-class” means that the rubbers have a saturated main chain of polymethylene type. Figure 2 shows the FTIR spectra of the black bulk rubber and the black residue on the fiber surface, as well as a library reference spectrum of EPDM. It should be noted that FTIR may not be able to differentiate between EPDM and EPM since the diene component is generally in low concentration. The spectra of the EPDM have CH2 asymmetric and symmetric stretch bands at 2923 cm-1 and 2851 cm-1. A CH2 scissors vibration at 1463 cm-1 is present, but it may include contribution from C-CH3 asymmetric bend. The spectra also have asymmetric CH3 stretches at 2954 cm-1 and a symmetric C-CH3 bend (umbrella mode) at 1377 cm-1. Similarly, the fiber and the brown residue were identified as Nylon 66 and SBR (styrene/butadiene rubber) respectively.
Identification of Unknown Particles in Formulated Drug Solution (Case 2)
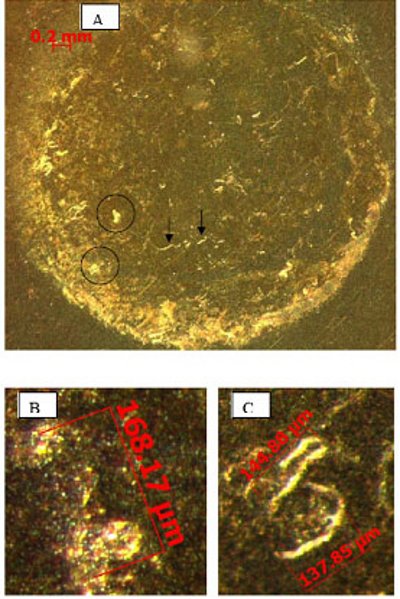
Figure 3 - (Case 2) A: overview of the particles on the gold coated filter. The representative irregular shape particles are indicated in circles and the representative fiber particles are indicated in arrows. B: an irregular shape particle in the larger magnification; and C: fiber-like particles in the larger magnification.
The particles were filtered onto a gold coated filter as shown in Figure 3A. Two representative types of particles were found on the filter. A typical irregular shaped particle with a size of 168 μm is shown in Figure 3B. Two typical fiber-like particles with sizes of 145 μm and 138 μm are shown in Figure 3C. The fiber particles are more condensed and have more defined contours. These two types of representative particles were further analyzed with FTIR-microscopy. The top hits with high HQI in this case did not provide meaningful clues in library searching. Examination was then focused on whether there are proteins in the spectra since these particles were found in a protein solution. Figure 4 shows the FTIR spectra of the irregular shape particle (blue trace) and the fiber particle (red trace). The spectra of the irregular and fiber particles look very similar to each other. Both spectra showed characteristic IR bands of protein: the amide I bands (C=O stretching mode) centered at 1629 cm-1, the N-H stretching mode centered at 3273 cm-1, and the amide II bands (C-N-H bending) centered at 1523 cm-1. The C=O stretching (1629 cm-1) of these particles is lower than native state of protein in liquid (1640 cm-1, data not shown). Lower wavenumber oTIR spectra of the bulk rubber and the black residue on fibers. A library reference of EPDM (Ethylene Propylene Diene Monomer) is also shown. The bulk rubber and the blacf C=O stretching means the protein in the solid particles has weaker C=O bonds than the native protein in liquid.
There were four additional strong sharp bands in the range of 1300-700 cm-1 in the FTIR spectra of both particles that are unlikely to be due to protein vibration. Limiting the range of the library search to 1300-700 cm-1 minimizes contributions of the protein and increases the impact of features in this specified region. This limited range search identified the second component as a type of silicone oil, PDMS (polydimethylsiloxane). In this range, the characteristic peaks of PDMS are the Si-CH3 bending (umbrella mode) at 1261 cm-1, the asymmetric stretches of Si-O-Si of siloxane chains, and the Si-O-C of side chains at ~1083 cm-1 and ~1013 cm-1. Also, there are two Si-C stretches at ~870 cm-1 and ~794 cm-1. Additionally, outside the searching range of 1300-700 cm-1, the spectra demonstrate a strong absorption for the asymmetric CH3 stretch at 2961 cm-1. Thus, both of the two representative particles were identified as a mixture of protein and silicone oil.
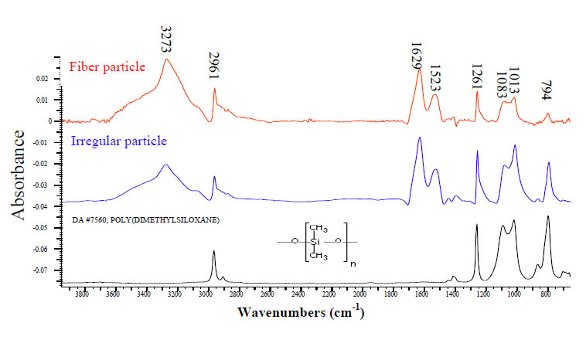
Figure 4 - (Case 2) FTIR spectra of the fiber particle and the irregular paritcle. They were identified as a mixture of protein and silicone oil. A library reference FTIR of silicone oil was also shown at the bottom.
Conclusions
For identification of unknown materials, FTIR has the advantage of being rapid, simple, and nondestructive; furthermore, it is able to directly analyze the samples without sample treatment or labeling. In this paper, two cases were presented to illustrate the important role of the combination of library searching and the experience of the spectroscopists. In Case 1, the power of FTIR coupled with light microscope was utilized to separately measure different heterogeneous compounds in the mixture, and library searching was applied separately for each different component. In Case 2, the spectroscopists used functional group analysis to identify the protein component, while the library searching was focused on the partial region of the spectrum to identify the silicone oil components. The two cases reflect the importance of selecting the most appropriate experimental technique and relevant library searching technique.
Acknowledgements
We are grateful to Dr. Linda Narhi and David Brems for reading the manuscript and to colleagues Gianni Torraca, Chanel Yee, Colin Cao, Aylin Vance and Peter Masatani in the Forensic Group for constructive discussions.
References
- G. Y. Li, , G. Torraca, , W. Jing, , Z. Q. Wen, Applications of FTIR in identification of foreign materials for biopharmaceutical clinical manufacturing, Vib. Spectrosc. 50 (2009)152-159.
- I. W. Evett, , P. D. Gill, G. Jackson, J. Whitaker, Interpreting small quantities of DNA: the hierarchy of propositions and the use of Bayesian networks. J Forensic Sci 47 (3), (2002) 520- 30.
- M. Hajimahmoodia, , Y. Vander Heydenb, N.Sadeghia, B. Jannata, M.R. Oveisia, S. Shahbazian, Gas-chromatographic fatty-acid fingerprints and partial least squares modeling as a basis for the simultaneous determination of edible oil mixtures. Talanta 66 (5), (2005) 1108-1116.
- Z. Stefanowics, J. Stefanowics, K. Mulas, Determination of Tropicamide And Its Major Impurity in Raw Material By the HPLC-DAD Analysis and Identification of This Impurity Using the Offline HPLC-FT-IR Coupling. J Pharm Biomed Anal 49(2), (2009) 214-220.
- W. A. Warr, Spectral Databases. Chemometr Intell Lab Syst 10 (1991) 279-292.
- J. P. Beauchaine, J. W. Peterman, R. J. Rosenthal, Applications of FT-IR/Microscopy in Forensic Analysis. Mikrochim Acta 1 (1988) 133-138.
- K. Laniewski, T. Wannman, G. Hagman, Gas Chromatography with Mass Spectrometric, Atomic Emission and Fourier Transform Infrared Spectroscopic Detection as Complementary Analytical Techniques For the Identification of Unknown Impurities in Pharmaceutical Analysis J Chromatogr. A 985, (2003) 275-282.
- G.Y. Li, M. Thompson, E. Dicarlo, A Chemometric Model for Determination of Cartilage Grade by Infrared Fiber Optic Probe Spectroscopy Appl Spectrosc, 59 (12), (2005) 1527-1533
- M. Beekes, P. Lasch, D. Naumann, Analytical Applications of Fourier Transfor-infrared (FT-IR) Spectroscopy in Microbiology and Prion Research. Vet Microbiol 123 (2007) 305-319.
- N. A. Ngo thi, C. Kirschner, D. Naumann, Characterization and Identification of Microorganisms by FT-IR Microspectrometry. J Mol Struct 661-662, (2003) 371-380.
- D. Naumann, Encyclopedia of Analytical Chemistry John Wiley & Sons, Chichester, UK, 2000
- M. R. Nyden, J. E. Pallister, D. T. Sparks, A. Salari, Computer-Assisted Spectroscopic Analysis Using Orthonormalized Reference Spectra. Part II: Application to the Identification of Pure Compounds. Appl Spectrosc 14 (1997) 9-17.
- M. Kunioka, F. Ninomiya, M. Funabashi, Novel Evaluation Method of Biodegradabilities For Oil-based Polycaprolactone. Polym Degrad Stabil, 92 (7), (2007) 1279-1288.
- D. J. Nagle, M. Celina, L. Rintoul, P. M. Fredericks, Infrared Microspectroscopic Study of the Thermo-oxidative Degradation of Hydroxy-terminated Polybutadiene/isophorone Diisocyanate Polyurethane Rubber. Polym Degrad Stabil 92 (8), (2007)1446-1454.
Author Biographies
Dr. Guiyang Li is a currently a scientist focusing on biopharmaceutical manufacturing incident investigation at the Forensic Lab at Amgen. He joined Amgen in 2005. He had been a postdoctoral researcher at the Hospital for Special Surgery, Cornell Medical School, New York. He obtained his Ph. D in Polymer Science from Case Western Reserve University, his M.S. in Polymer Science from Fudan University (China) and B.E. from Dalian University of Science of Technology (China). His recent interests lie in applying Chemometrics in vibrational spectroscopy to develop identity test methods for raw materials and quantitative methods in critical process characterizations in biopharmaceutical fields.
Mrs. Wen Jing is currently a senior associate scientist working at Forensic Lab at Amgen in manufacturing incident investigation. She joined Amgen in 2005. Prior to Amgen, she had worked as a research chemist in polymer materials area almost 20 years. She obtained a M.S. in Science of Chemistry Major in Polymer from Eastern Michigan University, Ypsilanti, MI. and B.E. in Polymer Technology from East China University of Chemical Technology, Shanghai, P.R.China. Her interests include forensic identification of particles during the biopharmaceutical manufacturing processes and quality control of final products, elucidation of polymers used in biopharmaceutical industries.
Dr. Zai-Qing Wen is currently a principal scientist working at the Forensic and Biophysical Analysis Lab at Amgen Inc. He joined Amgen in 1999. Prior to Amgen, he had worked as research instructor at The University of Missouri at Kansas City and as a Visiting Scholar at The Protein Research Institute, Osaka University, Japan. He obtained his Ph.D. in Biophysical Chemistry from The Glasgow University, U.K., and a BS in Chemical Physics from The University of Sciences and Technology of China. Dr Wen’s interests include vibrational spectroscopy (FTIR and Raman, UVRR and ROA) and their application to protein pharmaceutical structure characterization and polymer material characterization. Dr. Wen has authored over 40 publications.
This article was printed in the November/December 2011 issue of American Pharmaceutical Review - Volume 14, Issue 7. Copyright rests with the publisher. For more information about American Pharmaceutical Review and to read similar articles, visit www.americanpharmaceuticalreview.com and subscribe for free.