Ping Liu - Associate Director, Vector Core-Cell Line Development, REGENXBIO Inc.
Ayda Mayer - Executive Director, Vector Core, REGENXBIO Inc.
Abstract
Recombinant Adeno-Associated Virus (rAAV) is the leading vehicle for delivery of gene products for the treatment of gene therapy. Low production yields and product quality contribute to high manufacturing costs which remain a key challenge of the field to date. In this review, we summarize the recent advances in the commonly used platforms including HEK293, Baculovirus, HSV, and HeLa based methods for rAAV production. The advantages and major challenges of each method and strategies to improve production yields and product quality are addressed in detail.
Introduction
rAAV based gene therapy has gained a lot of attention in recent years as it has several desirable attributes including lack of pathogenicity and low immunogenicity.1 rAAV-based vectors target both dividing and non-dividing cells including retina, liver, heart, muscle, and Central Nervous System (CNS) to allow for long-term expression of therapeutic genes.2 To date, more than 200 clinical trials have been conducted for a variety of diseases such as hemophilia A and B, Parkinson’s disease, wet age-related macular degeneration, Mucopolysaccharidosis (MPS), Batten disease, and many others.3 In 2012, alipogene tiparvovec, developed to treat reverse Lipoprotein Lipase Deficiency (LPLD), became the first approved gene therapy drug in Europe.4 Since then, two other gene therapy products have been approved by the U.S. Food and Drug Administration (FDA) including the approval of voretigene neparvovec to treat Leber congenital amaurosis in 20175 and onasemnogene abeparvovec to treat Spinal Muscular Atrophy (SMA) in 2019.6
Despite the success of clinal trials for rAAV based gene therapy, high manufacturing cost remains a bottleneck for the gene therapy field due to low production yields and high dose demands for certain disease indications. Onasemnogene abeparvovec, with a cost of $2.1 million for a single dose treatment, is currently the most expensive drug in the US market. As another example, treating X-Linked Myotubular Myopathy (XLMTM) requires 1014 vector genomes per kilogram (vg/kg) and at least 1015 vg/patient.3 Although recent process and technology developments have improved production yields to 1011 to 1012 vg/L and reduced the cost of goods,7 the upstream production yields are still not high enough to satisfy the large dose requirement for diseases such as XLMTM.
Adeno-Associated Virus Biology
Wild-type Adeno-Associated Virus (AAV) was accidentally discovered in 1965 by Atchison et al. as a small contaminating particle of 25 nm in adenovirus preparations for electron microscopy.8 It required adenovirus for replication and later was classified to the Parvoviridae family of non-enveloped viruses.9 Wild-type AAV contains a single stranded DNA genome of 4.7 kb that spans two main open reading frames (ORFs) flanked by two T-shaped inverted terminal repeat sequences (ITRs) (Figure 1).10 The inverted terminal repeats have replication protein binding elements (RBE), and terminal resolution sites (TRS), which are involved in replication, genome packaging in the capsid, and long-term intracellular persistence.11,12 The first open reading frame (rep) contains P5 and P19 promoters and encodes four replication proteins through alternative splicing (named Rep78, Rep68, Rep52, and Rep40 after their molecular weights) (Figure 1). Rep78 and Rep68 expression are regulated by the P5 promoter. They are required for DNA replication, site-specific integration, regulation of gene expression, and excision from the host genome.13 The P19 promoter regulates Rep52 and Rep40 expression. Both Rep52 and Rep40 have 3’ to 5’ helicase activity and are important for AAV genome packaging.14,15 The second open reading frame (cap) contains P40, a promoter which controls the expression of three capsid protein subunits, namely VP1, VP2, and VP3, with molecular weights of 87, 72, and 62 kDa, respectively. The carboxy-terminus protein sequence is identical for VP1, VP2, and VP3.
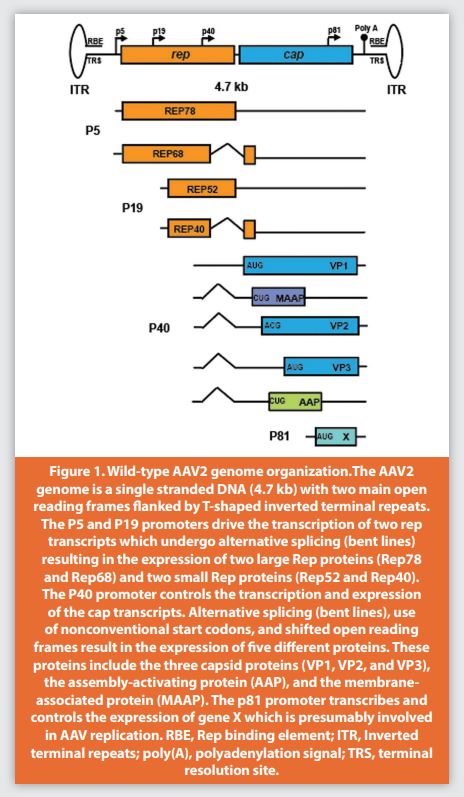
The capsid proteins are produced in a 1:1:10 ratio (VP1:VP2:VP3) from two alternatively spliced mRNAs that assemble a capsid made of 60 subunits of viral protein. The minor spliced RNA variant from the P40 promoter transcript contains the VP1 translational start codon (AUG) which explains its lower expression levels compared to VP3. While the major spliced RNA variant contains translational initiation sites for VP2 and VP3, the translation of the latter is initiated from a conventional AUG start codon, whereas the former is produced from a nonconventional ACG start site that is frequently skipped and results in lower VP2 expression (Figure 1).12 The N-terminus of VP1 also contains enzymatic activity represented by phospholipase A2 (PLA2) which is essential for AAV escape from endosomes and contains the nuclear localization signal (NLS).16,17 The cap region also contains a frame-shifted open reading frame that encodes a nonconventional codon CUG for the assembly-activating protein (AAP) which plays an important role in most AAV serotypes18 during the transport of the VP subunit proteins to the nucleolar compartment and capsid assembly.19 The AAP protein is reported to interact with the conserved C-terminus of the capsid’s protein and function as an assembly scaffold.20 An additional two open reading frames were identified in the cap region that encode for protein X and the membrane associated accessory protein (MAAP). Although their precise functions in AAV biology are still unclear, they might be involved in the enhancement of AAV replication and secretion, respectively (Figure 1).21,22,23 An rAAV only contains the ITR from the wild-type virus, while the essential elements for replication and encapsidation (rep and cap) are replaced by the expression cassette for the gene of interest.
Current Recombinant Adeno-Associated Virus (rAAV) Production Platforms
Five production platforms are discussed in this review. Each has its own advantages and disadvantages in terms of rAAV quality, yield, speed of implementation, and reliable scalability. One of the most widely used platforms is the transient transfection of HEK293 cells for rAAV production. The other commonly used platforms include the Baculovirus expression vector system (BEVS) and the HeLa cell-based system, mainly because of their straightforward scalability for large volume production.24,25 Another well-known system is based on Herpes Simplex Virus (HSV) which also provides direct scalability for rAAV production.26 The final platform involves stable cell line development using HEK293 cells or other cell types in a helper virus free system, without using adenovirus, BEVS, HeLa, or HSV in production; this has become a hot topic lately and has made substantial progress.27
To overcome the bottlenecks faced in current rAAV production platforms, several research groups have explored the feasibility of using alternative hosts for rAAV production such as Escherichia coli and Saccharomyces cerevisiae, which are known to have well-established protein production platforms with a lower cost of goods. A recent study showed that AAV2 VP3 capsid subunits were expressed in E. coli and assembled successfully, with similar physical and biochemical properties to rAAV2 produced in HEK293 by a transient transfection process.28 Because of the lack of proper protein folding and post-translational modifications, other research groups explored the use of Saccharomyces cerevisiae as another alternative to produce rAAV with a simplified downstream process.29 Using four plasmids that encode for Rep78, Rep52, capsid VP1, VP2, VP3, and AAP to transform yeast cells, the research groups observed proper assembly of the rAAV but with lower % full particles and infectivity than rAAV produced by the more developed Sf9/baculovirus system.30 Future studies in yeast systems that focus on identifying the yeast host factors involved in capsid assembly, AAV replication, and packaging will aid in developing a better yeast system that is more scalable and cost-effective for AAV production.
HEK293-Based rAAV Production System
rAAV was first produced by transient transfection with three plasmids which carry the essential genes for rAAV assembly in HEK293 or HEK293T host cells that both stably express the transfected DNA of adenovirus E1A and E1B genes, supporting AAV replication.31 Although HEK293T cells have a faster growth rate and better transfectability with higher production for rAAV compared to HEK293 cells,32 the presence of the SV40 T-antigen oncoprotein presents a safety risk. The encoded protein from E1A increases rep expression by transactivating the P5 and P19 promoters that then initiate AAV replication. Supplying the rest of the essential adenovirus helper genes (E4, E2A, and viral associated (VA) RNAs) on one plasmid, in addition to a second plasmid encoding for rep and cap, and a third plasmid containing an expression cassette flanked by two inverted terminal repeats (ITRs), prevents adenovirus contamination during rAAV production (Figure 2A).33
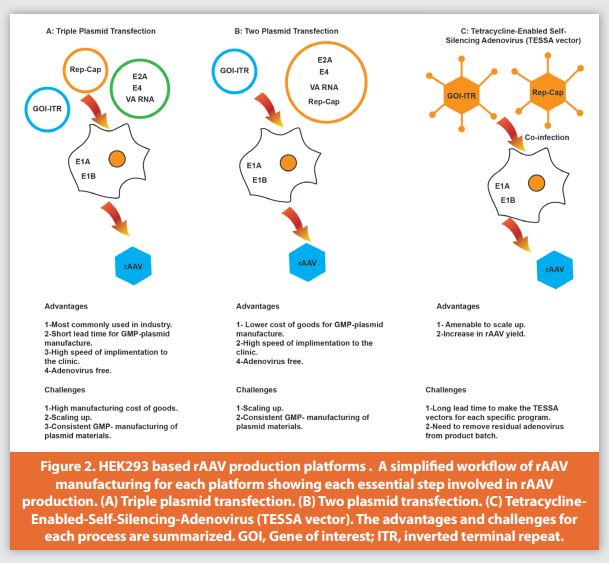
Traditionally, adherent HEK293 cells were used in the triple transfection plasmid method for rAAV production, this generated sufficient rAAV for initial clinical trials.25,34 Several advances and novel approaches were developed to move away from adherent HEK293 cells to serum-free suspension. This adaptation circumvented the difficulty of scaling adherent transient transfection technology.35-37
The adeno-associated virus is a dependoparvovirus and requires several host factors and the essential genes provided by a helper virus to replicate.38 Several helper viruses in addition to the adenovirus were identified and characterized to support propagation, such as herpes simplex virus, vaccinia virus in mammalian cells, and baculovirus in insect cells.39 Wang et al. discovered that human bocavirus 1 (HBoV1) was a novel helper virus for AAV2 replication, and the minimum requirement for AAV2 replication included HBoV1, NP1, and NS4 nonstructural proteins, and the viral long noncoding RNA (BocaSR).40 A subsequent study by the same team showed that combining the human bocavirus helper genes (NP1 and NS4) with adenovirus helper essential genes (E4, E2A, and VA RNA) gave roughly two-fold higher production yield than using the adenovirus helper plasmid alone in the triple transient transfection method.41
The triple plasmid transfection system is well established and commonly used for clinical and commercial manufacturing. Several research groups tried to introduce a two-plasmid system to shorten the time and lower the cost of goods needed to manufacture GMP product, in addition to simplifying the process of transient transfection (Figure 2B). The first two-plasmid expression system was introduced by Grimm et al., in which they designed a novel packaging/helper plasmid pDG to include the rep, cap, E4, E2A, and VA RNA genes that are required for AAVreplication, capsid formation, and helper functions. In this plasmid, the p5 promoter was replaced by an inducible Mouse Mammary Tumor Virus (MMTV) promoter to weaken Rep protein expression. Co-transfection of cells with the pDG plasmid and the transgene vector plasmid was sufficient for AAV production. The authors reported a 10-fold higher AAV titer produced by this two-plasmid system than by the conventional triple transfection system.42 In a similar approach, Tang et al. made further modifications to the backbone of the pDG plasmid to create a pQT packaging plasmid that contains AAV rep and cap, in addition to the essential adenovirus helper genes needed for rAAV production. They compared the pQT system to the traditional three-plasmid system using different AAV serotypes including AAV1, AAV5, AAV8, and AAV9 and found that the pQT system functioned similarly to the triple transfection system and was more flexible in terms of speed and cost effectiveness for GMP manufacturing.43
Recently, Su et al. introduced an elegant design for a self-attenuating adenoviral system suitable for scale-up that resulted in a 10-to-30-fold increase in rAAV production over a range of serotypes when compared to the traditional transient transfection method (Figure 2C).44 In this system, the researchers were able to control the helper adenovirus life cycle by turning on/off early and late temporal phases which are responsible for the helper functionality for rAAV production and adenovirus structural proteins expression, respectively. This was achieved by engineering the adenovirus Major Late Promoter (MLP) by insertion of tetracycline operator TetO that can be repressed with TetR repressor expressed from the major late promoter. This design created a negative feedback loop to regulate the expression of adenovirus structural genes controlled by the presence and absence of doxycycline. rAAV production is achieved by infecting HEK293 cells with a Tetracycline-Enabled Self-Silencing Adenovirus (TESSA vector) that provides expression of early genes with helper function in which the AAV transgene flanked with ITR is stably incorporated, and a second TESSA vector containing AAV rep and cap genes in the same region. Although this system showed increased rAAV yield over the conventional triple plasmid transfection and is amenable to scaling, there is still a very low level of adenoviral contamination. This is presumably due to the inherent leakiness of the doxycycline regulated promoter.45 Furthermore, it has been shown that one adenovirus fiber protein was expressed from a cryptic promoter at a very low level in the absence of the adenovirus Major Late Promoter (MLP).33 The complete clearance of residual adenovirus contamination can be achieved during downstream processing to ensure safety.
New technologies will further enable transient transfection scalability and improve rAAV quality, such as the use of plasmid-free transient transfection46 and nano plasmid platforms.47 In addition to implementation of plasmid-free platforms, others have explored new transfection reagents that support high transfection efficiency and high productivity in large scale bioreactors.48
Baculovirus-Based Expression System
The baculovirus expression system is an infection-based system. It is highly amenable to scaling for larger volumes, and utilizes a non-mammalian insect cell such as Spodoptera frugiperda (Sf9) that can grow in suspension and serum free medium. Furthermore, the baculovirus itself has helper functions equivalent to the adenovirus helper functions required for rAAV replication. The first expression system was the Three-Bac system developed by Urabe et al. (Figure 3A).49 In this system, Sf9 cells were infected with three different baculoviruses: Bac-Rep, expressing Rep78 under the control of a truncated early promoter E1(Δ1E1) from the Orgyia pseudotsugata nuclear polyhedrosis virus and Rep52 under the control of the baculoviral p10 promoter, in a head-to-head orientation; Bac-VP, expressing capsid proteins (VP1, VP2, and VP3) under the late polyhedrin (polh) promoter with the splicing intron removed due to the inefficient splicing machinery of Sf9; and Bac-GFP, expressing the gene of interest flanked by the AAV inverted terminal repeat (ITR) elements. To ensure the AAV capsids were generated in Sf9 cells with a similar stoichiometric ratio to those produced by mammalian cells, the start codon ATG of the VP1 gene was mutated to ACG to weaken VP1 expression in Sf9 cells. In this study, AAV vector produced in insect cells had similar physical and biochemical properties to those produced in mammalian cells. The virus titer yield reached 5×104 vg/cell.49 Thus, a 1 L culture of Sf9 cells is equivalent to 500 to 1000 T-175 shake flasks of HEK293. Despite these promising results, this first generation baculovirus system had a major drawback: instability of Bac-Rep due to homologous recombination events between rep52 and rep78, since they share identical overlapping sequences. This manifested as a titer decrease after a few passages.50 Furthermore, the expression level of the VP1 protein important for rAAV infectivity was not sufficient.17
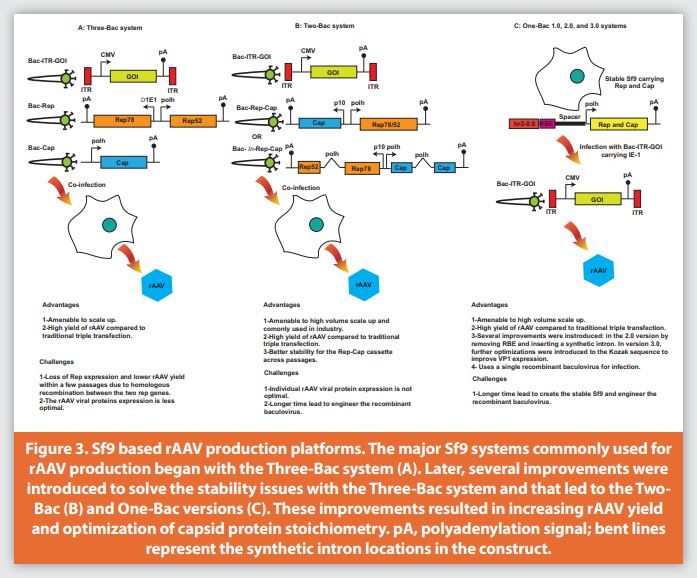
To overcome the challenges of the Three-Bac system, several research groups tried independently to resolve the instability issue and improve VP1 expression using two different approaches. In the first, the Rep and Cap vectors were further modified to generate a Two-Bac system (Figure 3B) as described by Smith et al.51 Rep78 and Rep52 were expressed from a single mRNA species by a “leaky scanning mechanism” without duplication of the rep coding sequences under the control of the baculovirus polyhedrin promoter. In addition, the start codon of Rep78 was mutated to a weak translation initiation codon, CTG, and codon optimization was performed by changing the next nine in-frame ATGs into non-start codons while retaining the Rep52 start codon as ATG.51 When this suboptimal translation initiation signal is encountered, a portion of the ribosomal subunits initiate translation at this site, while the remaining 40S subunits continue to search for the next translational initiation site. This resulted in the expression of full length Rep78 and a truncated protein sequence of Rep52. In the case of the cap gene, its expression was driven by the baculovirus p10 promoter with start codon ACG. Both rep and cap genes were placed in a head-to-head orientation in one baculovirus construct while the second virus carried the transgene flanked by ITR. Virus titer yield reached 7×104 vg/cell, although the expression level of VP1 was still lower than that produced from transient transfection systems.52
In the second approach, Chen introduced an artificial intron that carries the polyhedrin promoter into the rep78 coding sequence at the p19 promoter region in order to allow the expression of both Rep78 and Rep52 from a single coding sequence, this disrupted the homologous regions between Rep78/52 and the Three-Bac system was kept as is (Figure 3B).53 Similarly, an intron with a polyhedrin promoter was introduced into the capsid coding sequence to weaken the VP1 protein expression while maintaining the stoichiometry produced from the transient transfection system. Furthermore, the start codon for VP1 was kept as ATG which resulted in an rAAV product with two-fold higher infectivity compared to the case with an ACG start codon. Improvement in titer was observed for rAAV serotypes 1, 2, 6, and 8, and the yield ranged from 3.53×1013 to 1.58×1014 vg/L culture (Figure 3B).54
Further improvements were made for the Two-Bac system by simply designing a single baculovirus system, this was the basis of the Mono-Bac system in which one baculovirus carries all the necessary rep and cap genes (Bac-rep-cap) under the control of the late polyhedrin and p10 promoters, respectively, in a head-to-head orientation. This cassette was integrated into the dispensable egt (ecdysteroid UDP-glucosyltransferase) locus of the baculovirus genome by homologous recombination while the transgene flanked by the ITR was transposed to the attTn7 site by Tn7 transposase.55
The One-Bac system was introduced by Aslanidi et al. (Figure 3C).56 In this system, a producer Sf9 cell line was generated by stably integrating the rep and cap genes, each controlled by a polyhedrin promoter, and the enhancer element hr2-0.9. The rep and cap gene cassettes utilized in the producer Sf9 were constructed in an identical fashion, where each contained the enhancer element hr2-0.9 followed by an AAV rep binding element (RBE), a stuffer sequence, and a polyhedrin promoter that controlled expression. In the producer Sf9, expression of the rep and cap genes remained silent until cells were infected with the baculovirus carrying the transgene flanked by inverted terminal repeats and the immediate-early (IE-1) transcriptional trans-regulator that binds to the hr2- 0.9 target sequence. The binding of the trans-regulator to hr2-0.9 induced rep and cap expression and amplification. This resulted in up to 1200 copies per cell and a 10-fold increase in rAAV production in comparison to the original baculovirus system.49,56
The One-Bac system was further developed into the second generation One-Bac 2.0 by Mietzsch et al. in which the RBE were removed from the rep and cap gene cassettes (Figure 3C).57 This modification led to a still functional system with less amplification of the integrated rep and cap genes and less encapsidation of these genes in the final rAAV production. Additional modifications for this system were made by switching to the original start codon for VP1 (ATG) instead of (ACG) and insertion of an intron into the cap gene containing a polyhedrin promoter.53 These modifications resulted in increased VP1 expression and a subsequent increase in AAV5 vector infectivity in comparison to AAV5 produced using the original One-Bac system which showed a lower level of VP1 expression.58 The third generation One-Bac 3.0 further optimized the stoichiometry of VP by other modifications to the translational initiation codon and Kozak sequences (Figure 3C). This resulted in optimal VP1 expression levels in both AAV5 and AAV9, with high infectivity.59 Generation of the stable Sf9 cells upon which the One-Bac system relies is more technically challenging in insect cells. Therefore, the commonly used system is the Two-Bac, combining the simplicity of the One-Bac or Mono-Bac with relative stability compared to the Three-Bac.
Recently, a high producer clone, ExpiSf9, was generated from parental Sf9 cells and evaluated for production of three rAAV serotypes. This clonal cell line demonstrated 7-, 15-, and 19-fold increase in the yields for AAV9, AAV2, and AAV8, respectively, over the parental Sf9. On the other hand, the results of the in vivo transduction efficiency for the insect cell produced AAV9 were lower than that from the mammalian produced AAV9 due to the inherent suboptimal capsid VP1 ratio.60
There are still some limitations for the baculovirus system despite the recent improvements. The baculovirus stoichiometric ratio of VP1, VP2, and VP3 is still different from mammalian produced rAAV and more work needs to be done to identify the essential baculovirus helper genes required for rAAV production.61 Furthermore, the differences in capsid protein post-translational modification profiles between mammalian and insect cells, their negative impact on rAAV infectivity,62 maintaining long term baculovirus stock supplies, and the instability issues across increased passages will need to be addressed.63
HeLa-Based Expression System
The first-generation HeLa-derived stable packaging and producer cell lines were generated by Clark et al.64 These stable cells originated from transfection of HeLa cells with a plasmid containing both the rep-cap genes from AAV2 and an rAAV vector genome with a drug selection marker. The system was further developed by several groups to introduce a packaging cell line containing the Rep and Cap functions of AAV as an intermediate step. Additional packaging cell lines were created from HeLa cells65-67 or from A549 cells68 which were derived from human lung alveolar cell carcinoma without viral transformation.69 The cell lines used in making these stable packaging cells lacked adenovirus E1A/E1B genes, which are necessary to transactivate p5, p19, and p40 for transcription of rep and cap genes. Therefore, they must first be infected with an adenovirus that provides these functional genes (E1A/E1B), and then subsequently infected with AAV-hybrid virus (Ad-AAV Hyb.virus), an adenovirus that contains the rAAV transgene sequence flanked by ITR in the E1 region of the adenovirus.70 Use of a replication defective adenovirus is preferred to avoid replication and subsequent contamination of the final rAAV product. Purification protocols were developed to remove adenovirus during production (Figure 4).
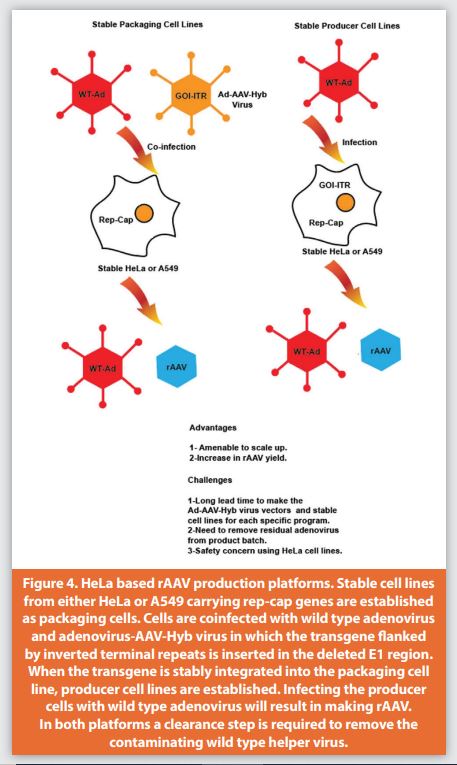
One replication defective adenovirus had a temperature sensitive mutation in the E2B gene which allowed virus replication only at the permissible temperature. The drawbacks of the temperature sensitive mutant were that it reverted to wild type and was difficult to produce, and subsequently it could not be easily implemented for large scale production.71 A HeLa-based stable cell line was used for production of the first rAAV product administered to humans in a clinical trial for the treatment of cystic fi brosis.72 The major advantage of the system was the lack of transfection. The rAAV production relied on infection of the cells with adenovirus and the system could be directly scaled up if there was an established protocol to remove the contaminating adenovirus and viral proteins from the final rAAV product.73 The disadvantages of this system are the long lead time needed to establish stable packaging/producer cell lines, and GMP stock generation for the adenovirus vectors. Additionally, the use of the HeLa cell line has some safety concerns since it is known to contain multiple copies of several oncogenes encoding for the human papillomavirus early proteins.74
HSV Type 1-Based Expression System
Herpes Simplex Virus type 1 (HSV-1) provides helper functions for AAV replication and has been studied as extensively as adenovirus.75 In comparison to adenovirus, HSV-1 replication proteins are involved directly in rAAV replication, and it has a genome of 152 kb of double stranded DNA that encodes for over 80 proteins. The genome is divided into two parts, known as unique long (UL) and unique short (US), that are flanked by inverted repeat regions.76
The essential genes in HSV-1, which induce AAV Rep expression and subsequently result in its replication, are described as the HSV-1 helicase-primase complex (HP) (UL5/UL8/UL52) and the single-strand DNA binding protein ICP8 (gene UL29).77,78 Other required helper genes not essential for replication but reported to support AAV Rep expression include ICP0 (E3 ubiquitin ligase, a trans-activator of HSV-1 gene expression), ICP4 (a major viral transcription factor), and ICP22 (a transcriptional regulator). Furthermore, UL30 and UL42 are HSV-1 polymerase catalytic subunits that directly enhance AAV genome replication.79 Although it has been shown that the four HSV-1 proteins (UL5/UL8/UL52 and ICP8) are sufficient to restore AAV DNA replication, the replication efficiency is very low compared to what has been observed in the presence of all HSV-1 genes. Other unknown HSV-1 factors are required to fully activate rAAV replication.80 In fact, it has been recently shown by Meier et al. that HSV-1 supported rAAV genome replication uses a rolling circle replication (RCR) mechanism instead of a strand-displacement rolling hairpin replication (RHR) mechanism as documented for adenovirus supported replication.81
Conway et al. reported the first-generation method for rAAV production based on the HSV amplicon system.82 In this system, the AAV2 rep and cap cassettes were cloned into a plasmid carrying the HSV origin of replication and packaging signals. To produce HSV virus carrying the AAV2 rep and cap genes, this plasmid was transfected into Vero cells that were pre-infected with wild-type HSV-1 to generate HSV carrying AAV2 rep and cap genes (HSV-RC). The helper functions were supplied in trans from the pre-infection with wild-type HSV-1. The HSV-RC virus produced was then used to infect HEK293 carrying an integrated rAAV cassette or transfected with a plasmid carrying the transgene flanked by AAV2 ITR or infected with an rAAV2 virus.
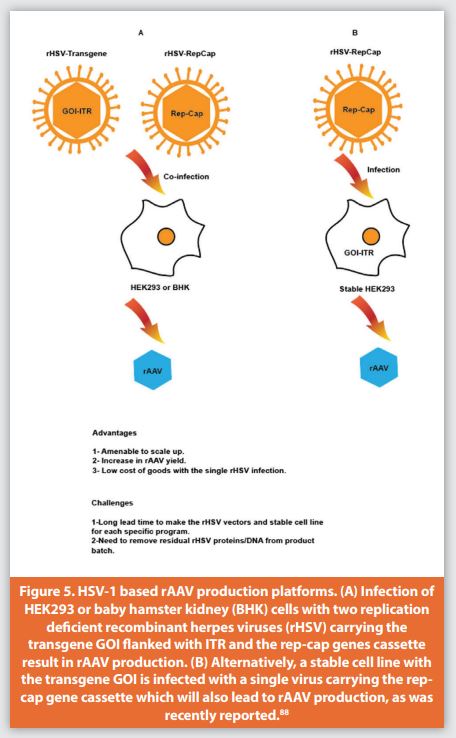
Although this approach resulted in rAAV, the yield was low and there was a gradual loss of the HSV-RC across several passages. Furthermore, the use of the wild-type HSV-1 was undesirable due to safety concerns, in addition to its cytopathic effect and subsequently lower rAAV yield. Removing wild-type or other live virus generated in the upstream process requires robust purification processes, which frequently result in significant yield loss and may also have issues with scale-up. Further improvements to this method were introduced by the same group upon the generation of an HSV mutant with the deletion of the ICP27 encoding gene (UL54), which caused the observed cytopathic effect. These changes significantly reduced cytotoxicity and increased the yield of rAAV.
The second-generation method was also introduced by Conway et al., in which the amplicon was replaced by a recombinant HSV (rHSV) infection (Figure 5A).83 The AAV-2 rep-cap cassette was inserted into the Thymidine Kinase (TK) locus of ICP27-deficient HSV by homologous recombination and was propagated in V27 cells to produce the rHSV virus.84 V27 cells are a derivative of Vero cells that stably carry the UL54 gene which encodes for ICP27 protein supplied in trans to generate the rHSV. These improvements gave a higher rAAV yield after using the rHSV to infect HEK293 cells that were stably carrying the rAAV transgene cassette, or transfected with a plasmid containing the rAAV transgene cassette, or infected with an rAAV virus. Furthermore, the cytopathic effect (ICP27 protein) was prevented, and both the HSV-1 helper functions and rep-cap genes were delivered on a single infectious vector. This system was developed using adherent cells and still relied on transfection of a plasmid, which introduced some limitations.
The next step for this platform development was to move away from adherent cells and implement a transfection free system. Hwang et al. used the ICP27-deficient HSV-1 to generate two rHSV vectors carrying the transgene flanked by AAV2 ITR and AAV-rep-cap cassettes (Figure 5A).85 The cassettes were inserted by homologous recombination into the Thymidine Kinase (TK) locus. The two rHSV vectors were used to coinfect HEK293 cells in culture flasks. A significant increase in rAAV yield was observed relative to transient transfection in HEK293 cells or to single rHSV infection. Using Baby Hamster Kidney cells (BHK) adapted to grow in suspension serum free culture allowed a lower Multiplicity Of Infection (MOI) and circumvented the slight toxicity of the Rep protein. In addition, BHK cells had the advantage of faster growth and high infectivity compared to HEK293 cells. This subsequently led to the use of a lower MOI during co-infection.71 Recently, two groups have looked at rAAV9 product yield and quality by comparing the rHSV and transient transfection-based platforms for adherent and suspension HEK293 cultures and reported that both methods had equal yield and potency for rAAV9 expressing micro-dystrophin.86 In the case of suspension Expi293F, the rHSV method gave better yield and a higher percentage of full capsids with less residual host cell protein and helper DNA, while higher HSV DNA was detected compared to the transient transfection suspension culture.87
Selvaraj et al. introduced further improvements to the rHSV-based method, where a stable cell line was developed from HEK293 that carries an rAAV transgene flanked with AAV2 ITR and a selection marker (Figure 5B).88 After selection for high rAAV producers using only one rHSV-rep-cap virus infection on adherent cells, they obtained a high yield of 1-2×1013 vg/6360 cm2 vessel with 50% full capsids in the final purification step. This approach could be adapted to suspension culture for scale up and certainly cut the cost of goods by making one rHSV-rep-cap virus instead of two. A well-established clearance protocol is needed in the downstream process to remove any residual HSV DNA or proteins in the final rAAV that could potentially induce an immune response in the host.89
Stable Cell Line Development for Recombinant AAV Production
The challenges of generating stable cell lines in HEK293 cells result from the endogenous expression of E1A that is stably integrated into the genome of HEK293. The expression of E1A could trans-activate rep and cap genes and turn on the helper genes such as E1B, E2A, and E4 as well. The early expression of Rep particularly is cytotoxic, which makes it very challenging to obtain a stable cell line. However, stable cell lines would be very beneficial to AAV production since they have several advantages including better scalability, less lot-to-lot variation, and lower cost of goods and better production yield. The first attempt to create a stable HEK293 cell line for rAAV production was by Yang et al.90 They replaced the p5 promoter with an inducible promoter to regulate the expression of Rep78/68. However, expression of the small Rep proteins (Rep52/40) was still occurring from the p19 promoter embedded in the overlapping open reading frames of the rep genes, therefore the inducible promoter did not result in the temporally coordinated expression as for the p5 and p19 promoters.90 In another elegant study by Qiao et al., they were able to control the expression of the four stably integrated cytotoxic Rep proteins (Rep78, Rep68, Rep52, and Rep40) by designing a dual splicing switch mechanism in the HEK293 cell line.91 In this system an intron cassette containing transcription termination sequences was inserted in the overlapping coding sequences of Rep proteins to prematurely terminate their expression without changing the P5 and P19 promoter activities. The expression of all four Rep proteins was then restored by infection with a defective adenovirus that carried Cre recombinase (Ad-Cre). Although they were able to obtain a high titer of rAAV using this approach, the undesirable aspect was the possible trace contamination with defective Ad-Cre in the rAAV product, which still presented small risks.
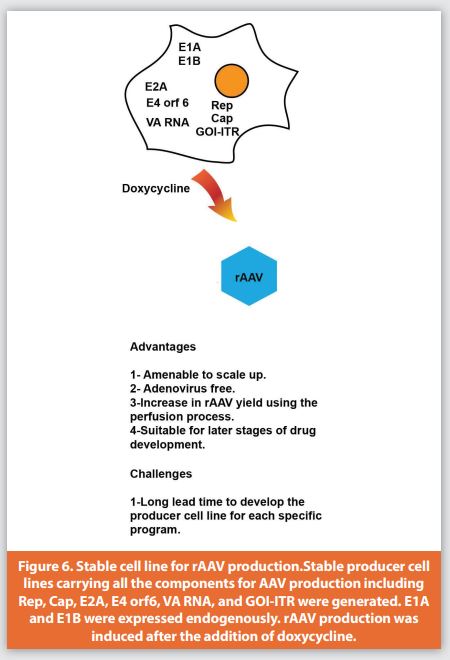
Recently, new stable AAV producer cell lines have been developed (Figure 6). In this technology, first packaging cell lines were generated to stably express Rep and the essential helper proteins, then subsequently the stable producer cells were generated by incorporating capsid and transgene plasmids into the packaging cell lines. The expression of rAAV particles was induced by doxycycline.92 This system offers many advantages to the field, such as eliminating the transient transfection step and being adenovirus-free and scalable as well. A yield of 1×1015 vg/L and more than 30% full particles for rAAV8 were achieved from the crude harvest using optimized processes in a perfusion bioreactor.27
Other Strategies for Improving rAAV Production Yield and Quality
Solving low rAAV genome packaging efficiency
During rAAV production, DNA packaging is considered the rate limiting step and product contains empty capsids without the therapeutic gene and the desired curative benefit.93,94 A recent, elegant study by Dash et al. showed that despite the 60% transfection efficiency, quantified by measuring GFP expression by flow cytometry following the transient transfection, only roughly 7% of the transfected cells contained fully assembled rAAV capsids.95 The assembled viral capsids were quantified using a conformation-specific antibody that binds only to assembled viral capsids. The triple plasmid transfection was compared to two positive controls including an infectious AAV2 clone with helper plasmid (26% full) and a replication competent AAV2 with adenovirus 5 (88% full). The study indicated that the low percentage of full particles by transient transfection was associated with the low level of capsid production, while the expression levels of helper protein such as E2A were not significantly different in transient transfection compared to cells infected with rcAAV and hAd5 virus. This study also suggested that AAV titers could be significantly improved by increasing the fraction of cells capable of assembling the rAAV since the low percentage of packaging is not correlated with transfection efficiency.95
A mechanistic model study that looked at plasmid uptake, Rep-Cap and helper protein expression, and rAAV assembly kinetics during triple plasmid transfection in HEK293 reported that the low percentage of full particles in the harvest was due to delayed DNA replication and early production of capsid.96 In fact, the model predicted that most of the capsids were assembled in the first 24 hours and capsid production was significantly reduced later. However, viral DNA replication starts later than capsid synthesis, around 12 hours, it peaks at 24 hours, and continues to remain steady for at least 48 hours. The study proposed two possible solutions to increase the percent of full capsids. The first is to enhance DNA replication in the early stages, which could be achieved by decoupling the expression of the Rep proteins from the capsid and delinking them from the same plasmid. This approach was previously reported by Emmerling et al.; their study used four-plasmid transfection by splitting the rep and cap genes and achieved higher rAAV yield with an increase in full capsids. The second solution relies on the temporal dosing of plasmids at different time points during transient transfection.
The AAV serotype 2 rep is used for all other AAV serotypes during rep-cap vector design to enable amplification and packaging of the single stranded DNA genome into capsids. A recent study by Mietzsch et al. tested the impact of using the corresponding rep gene of each serotype on genome packaging efficiency by replacing AAV2 rep with rep genes that corresponded to each serotype, and found the replacement resulted in lower capsid protein expression relative to the traditional AAV2-rep system, with lower packaging efficiency as well.98 However, Rep hybrids containing the 3’ end of the AAV-2 rep gene could improve full capsids two- to four-fold for AAV1, AAV8, AAV9, and AAVrh10. Furthermore, the authors did not find any differences in genome copy number and expression level of Rep52/40 proteins. This suggests that the Rep hybrids did not necessarily improve genome packaging by their small Rep52/40 since the sequences of the Rep52/40 are identical across the serotypes, with the exception of a single amino acid difference from AAV2-Rep (K234R). This finding further indicated that the large Rep proteins could be involved in genome packaging, contrary to what was commonly known,15 and the mechanism of genome packaging improvement for the Rep hybrids remains unknown. Additionally, the difference in nucleotide sequences among the rep genes of all AAV serotypes could result in the differential expression ratios of the Rep proteins by affecting promoter activities or mRNA splicing, and subsequently contribute to the increase in genome packaging efficiencies.98
Cell line engineering of host cells
rAAV production not only relies on direct helper functions but also on host cell factors which interact either indirectly with helper gene products or directly with rAAV and which ultimately affect rAAV yield.99 A limited number of studies have reported on developing or engineering cell lines for rAAV production. One of the early attempts to identify host factors that interact with rAAV during production was reported by Satkunanathan et al. using a proteomic analysis approach for three AAV serotypes, 2, 5, and 8, produced by transient transfection of HEK293T cells.100 The group identified 44 proteins associated with purified AAV particles using Liquid Chromatography-Mass Spectrometry/Mass Spectrometry (LC-MS/MS) methods; 10 proteins were shared among the three serotypes. They generated a novel cell line by knocking-down one of targets Y-box binding protein (YB1) using small hairpin RNA (shRNA) methods. The new engineering cell lines showed 45- and 9-fold titer improvement for AAV2 and AAV8, respectively, and a 7-fold increase in AAV2 transduction titers with no effect on AAV5. Further molecular analysis of the YB1 protein indicated that the knockdown cell line had 12- and 4-fold increases in AAV2-Rep protein expression at 24 and 72 hours after transient transfection, respectively, while it resulted in a 7-fold decrease in cap gene expression.100
Strasser et al. studied the host cell proteomic profiling of AAV5- producing HEK293 cells using reverse phase nano-liquid chromatography-tandem mass spectrometry (RPLC-MS/MS) and label-free quantification (LFQ).101 They compared the proteomic profiles after 72 hours for untransfected and transfected cells, pellets, and supernatants to study the effects of rAAV5 production on HEK293 cells. The authors found proteins involved in cell organization and biogenesis were strongly upregulated, while those proteins involved in cell cycle control and cell division were strongly downregulated. In this study, they utilized chloroquine, a known inhibitor that perturbs the endocytic pathway, to block endocytosis and endosomal acidification. The results indicated that adding chloroquine led to more than 35% improvement in AAV titers in cells and supernatant. This was attributed to blocking the rAAV5 uptake and trapping the newly produced rAAV5 in cells without degradation.101 The study clearly demonstrated the utility of using proteome profiling during rAAV production to target and perturb crucial signaling pathways to increase yield and maintain cell viability.
Barnes et al. conducted a genome-wide-screening study using a Cas9 activation approach to target the genes involved in rAAV production in HEK293T,102 which was subsequently validated in the HEK293 cell line. The single-guide RNA (sgRNA) pooled library for human CRISPR activation was introduced to HEK293T cells through several rounds of AAV infection, followed by rescue of the producer cells which enriched for the genes enhancing rAAV production. The top hits from their screen, including the spindle and kinetochore associated complex subunit 2 (SKA2), transcript variant 2, and inositol 1, 4, 5-trisphosphate receptor interacting protein (ITPRIP), were identified in this process. Overexpression of these genes led to a 4-fold titer increase in AAV2 and AAV6 production with a subsequent increase in full viral particles up to 2.4-fold. Both SKA2 and ITPRIP modulated the host cell cycle and enhanced rAAV genome replication, which is in line with the mechanistic model proposed by Nguyen et al. that predicted the importance of DNA replication for efficient viral genome loading into capsids.96
Process Development
Several parameters in the upstream cell culture process impact rAAV production yield and quality during transient transfection. These parameters include host cell lines, cell density at transfection, plasmids, plasmid/cell ratios, transfection reagents, media used for cell culture and transfection, incubation time during transfection, ratio of transfection medium to cell culture volume, feed media, and harvest time. Therefore, a slight change in each parameter will result in lot-to-lot variations. Furthermore, process optimization is challenging since many variables needed to be optimized and some variables interact. Adherent cells were initially used in the majority of HEK293 transient processes.33 Unfortunately, large scale production of adherent cells is very costly, and the rAAV productivity is very low as well. To improve the productivity and scalability, HEK293 cells were adapted from adherent to suspension culture. This change, in addition to selection for the highest producing clone, resulted in a titer of 1×105 vg/cell or 1×1014 vg/L in bioreactors.35,37,103 Perfusion culture has also been introduced in the AAV production process. In the study conducted by Grieger et al., a perfusion process in rocking motion bioreactors significantly improved viral titers.103 A recent study by Zhao et al. demonstrated that by using a design of experiments (DOE) approach they were able to evaluate multiple interdependent factors across 52 different conditions and optimize the AAV production in HEK293T cells to achieve 3×1014 vg/L of cell culture.7
Small chemical additives such as salts can also be used after transfection to boost viral titers and infectivity, Adamson-Small et al. showed that the addition of sodium chloride (NaCl) could improve titers in the herpes simplex virus system.104 Furthermore, Yu et al. also found that potassium chloride (KCl) or the combination of KCl and NaCl resulted in 10-fold higher AAV titers and an increase in viral infectivity.105 Neither group attributed the salt effect to changes in osmolality or cell growth as observed during monoclonal antibody production and reported previously.106 According to their observations, the salt supplementation was temporal and had a transient effect which appeared to correlate with the kinetics of virus production or cell cycle. They proposed that NaCl and KCl ion channels would be involved in this process while the exact mechanism remains to be fully identified. Furthermore, the salt effect is specific for the rHSV platform since they did not observe the same effect on rAAV produced by transient transfection.105
Concluding Remarks
It has been two decades since the initial use of AAV as mammalian vectors, and significant improvements have been achieved to advance AAV expression platforms.107 However, AAV platform development for gene therapy is still far away from the maturity reached in the antibody therapeutic field. There are several remaining bottlenecks and challenges to be solved in the future. For example, AAV production yields are still not satisfactory for diseases with high doses and large patient populations. Many AAV gene therapies still focus on the rare diseases with no existing efficient cure, which require only a low dose and have a small pool of patients. Improvement in the ratio of full to empty capsids, process yields, and potency are still hot topics in the field since empty viral capsids induce an immuno-response in patients potentially reducing the transduction efficiency and therapeutic impact. Furthermore, only a few research teams are actively working on engineering cell lines and plasmids to enhance titer and product quality. Stable cell line development for AAV production in HEK293 cells has attracted a lot of interest lately, however some technical issues still exist, for example endogenous E1A expression in HEK293 cells and the toxicity of Rep proteins make stable cell line development challenging. Besides the strategies discussed in this review, other efforts involving optimization of the promoters and transgenes in AAV vectors, and capsid engineering to improve tissue specificity and expression levels of therapeutic products, will be of great benefit.108 Pursuing innovative solutions could result in lowering the rAAV dose, reducing the yield requirement for the manufacturing process, and delivering safe and affordable gene therapy for patients.
Acknowledgment
The authors would like to thank Susan Wexelblat for editing the manuscript.
References
- Zaiss AK, Muruve DA. Immunity to adeno-associated virus vectors in animals and humans: a continued challenge. Gene Ther. 2008;15(11):808-816.
- Nonnenmacher M, Weber T. Intracellular transport of recombinant adeno-associated virus vectors. Gene Ther. 2012;19(6):649-658.
- U.S. National Library of Medicine. ClinicalTrials.gov. Accessed April 2022.
- Ylä-Herttuala S. Endgame: glybera finally recommended for approval as the first gene therapy drug in the European union. Mol Ther. 2012;20(10):1831-1832.
- Kumaran N, Michaelides M, Smith AJ, Ali RR, Bainbridge JWB. Retinal gene therapy. Br Med Bull. 2018;126(1):13 25.
- Hoy SM. Onasemnogene Abeparvovec: First Global Approval. Drugs. 2019;79(11):1255-1262.
- Zhao H, Lee KJ, Daris M, et al. Creation of a High-Yield AAV Vector Production Platform in Suspension Cells Using a Design-of-Experiment Approach. Mol Ther Methods Clin Dev. 2020;18:312-320. Published 2020 Jun 3.
- Atchison RW, Casto BC, Hammon WM. Adenovirus-associated defective virus particles. Science 149 (1965): 754-6.
- Cotmore SF, Agbandje-McKenna M, Canuti M, et al. ICTV Virus Taxonomy Profile: Parvoviridae. J Gen Virol. 2019;100(3):367-368.
- Rose JA, Berns KI, Hoggan MD, Koczot FJ. Evidence for a single-stranded adenovirus-associated virus genome: formation of a DNA density hybrid on release of viral DNA. Proc Natl Acad Sci U S A. 1969;64(3):863-869.
- Snyder RO, Im DS, Ni T, Xiao X, Samulski RJ, Muzyczka N. Features of the adeno-associated virus origin involved in substrate recognition by the viral Rep protein. J Virol. 1993;67(10):6096-6104.
- Weitzman MD, Linden RM. Adeno-associated virus biology. Methods Mol Biol. 2011;807:1- 23.
- Weitzman MD, Kyöstiö SR, Kotin RM, Owens RA. Adeno-associated virus (AAV) Rep proteins mediate complex formation between AAV DNA and its integration site in human DNA. Proc Natl Acad Sci U S A. 1994;91(13):5808-5812.
- Collaco RF, Kalman-Maltese V, Smith AD, Dignam JD, Trempe JP. A biochemical characterization of the adeno-associated virus Rep40 helicase. J Biol Chem. 2003;278(36):34011-34017.
- King JA, Dubielzig R, Grimm D, Kleinschmidt JA. DNA helicase-mediated packaging of adeno-associated virus type 2 genomes into preformed capsids. EMBO J. 2001;20(12):3282- 3291.
- Dhungel BP, Bailey CG, Rasko JEJ. Journey to the Center of the Cell: Tracing the Path of AAV Transduction. Trends Mol Med. 2021;27(2):172-184.
- Girod A, Wobus CE, Zádori Z, et al. The VP1 capsid protein of adeno-associated virus type 2 is carrying a phospholipase A2 domain required for virus infectivity. J Gen Virol. 2002;83(Pt 5):973-978.
- Earley LF, Powers JM, Adachi K, et al. Adeno-associated Virus (AAV) Assembly-Activating Protein Is Not an Essential Requirement for Capsid Assembly of AAV Serotypes 4, 5, and 11. J Virol. 2017;91(3):e01980-16. Published 2017 Jan 18.
- Sonntag F, Schmidt K, Kleinschmidt JA. A viral assembly factor promotes AAV2 capsid formation in the nucleolus. Proc Natl Acad Sci U S A. 2010;107(22):10220-10225.
- Naumer M, Sonntag F, Schmidt K, et al. Properties of the adeno-associated virus assembly[1]activating protein. J Virol. 2012;86(23):13038-13048.
- Cao M, You H, Hermonat PL. The X gene of adeno-associated virus 2 (AAV2) is involved in viral DNA replication. PLoS One. 2014;9(8):e104596. Published 2014 Aug 15.
- Hermonat PL, Santin AD, De Greve J, et al. Chromosomal latency and expression at map unit 96 of a wild-type plus adeno-associated virus (AAV)/Neo vector and identification of p81, a new AAV transcriptional promoter. J Hum Virol. 1999;2(6):359-368.
- Ogden PJ, Kelsic ED, Sinai S, Church GM. Comprehensive AAV capsid fitness landscape reveals a viral gene and enables machine-guided design. Science. 2019;366(6469):1139- 1143.
- Thorne BA, Takeya RK, Peluso RW. Manufacturing recombinant adeno-associated viral vectors from producer cell clones. Hum Gene Ther. 2009;20(7):707-714.
- van der Loo JC, Wright JF. Progress and challenges in viral vector manufacturing. Hum Mol Genet. 2016;25(R1):R42-R52.
- Clément N, Knop DR, Byrne BJ. Large-scale adeno-associated viral vector production using a herpesvirus-based system enables manufacturing for clinical studies. Hum Gene Ther. 2009;20(8):796-806.
- Coronel J, Patil A, Al-Dali A, Brab T, Faust N, Wissing S, Efficient production of rAAV in a perfusion bioreactor using an ELEVECTA® stable producer cell Line. Genetic Engineering & Biotechnology News 41, No 4. 2021: S23.
- Le DT, Radukic MT, Müller KM. Adeno-associated virus capsid protein expression in Escherichia coli and chemically defined capsid assembly. Sci Rep. 2019;9(1):18631. Published 2019 Dec 9.
- Galli A, Della Latta V, Bologna C, et al. Strategies to optimize capsid protein expression and single-stranded DNA formation of adeno-associated virus in Saccharomyces cerevisiae. J Appl Microbiol. 2017;123(2):414-428.
- Barajas D, Aponte-Ubillus JJ, Akeefe H, Cinek T, Peltier J, Gold D. Generation of infectious recombinant Adeno-associated virus in Saccharomyces cerevisiae. PLoS One. 2017;12(3):e0173010. Published 2017 Mar 29.
- Graham FL, Smiley J, Russell WC, Nairn R. Characteristics of a human cell line transformed by DNA from human adenovirus type 5. J Gen Virol. 1977;36(1):59-74.
- Stacey GN, Merten OW. Host cells and cell banking. Methods Mol Biol. 2011;737:45-88.
- Xiao X, Li J, Samulski RJ. Production of high-titer recombinant adeno-associated virus vectors in the absence of helper adenovirus. J Virol. 1998;72(3):2224-2232.
- Matsushita T, Elliger S, Elliger C, et al. Adeno-associated virus vectors can be efficiently produced without helper virus. Gene Ther. 1998;5(7):938-945.
- Durocher Y, Pham PL, St-Laurent G, et al. Scalable serum-free production of recombinant adeno-associated virus type 2 by transfection of 293 suspension cells. J Virol Methods. 2007;144(1-2):32-40.
- Hildinger M, Baldi L, Stettler M, Wurm FM. High-titer, serum-free production of adeno-associated virus vectors by polyethyleneimine-mediated plasmid transfection in mammalian suspension cells. Biotechnol Lett. 2007;29(11):1713-1721.
- Park JY, Lim BP, Lee K, Kim YG, Jo EC. Scalable production of adeno-associated virus type 2 vectors via suspension transfection. Biotechnol Bioeng. 2006;94(3):416-430.
- Maurer AC, Weitzman MD. Adeno-Associated Virus Genome Interactions Important for Vector Production and Transduction. Hum Gene Ther. 2020;31(9-10):499-511.
- Geoffroy MC, Salvetti A. Helper functions required for wild type and recombinant adeno-associated virus growth. Curr Gene Ther. 2005;5(3):265-271.
- Wang Z, Deng X, Zou W, Engelhardt JF, Yan Z, Qiu J. Human Bocavirus 1 Is a Novel Helper for Adeno-associated Virus Replication. J Virol. 2017;91(18):e00710-17. Published 2017 Aug 24.
- Wang Z, Cheng F, Engelhardt JF, Yan Z, Qiu J. Development of a Novel Recombinant Adeno-Associated Virus Production System Using Human Bocavirus 1 Helper Genes. Mol Ther Methods Clin Dev. 2018;11:40-51. Published 2018 Oct 4.
- Grimm D, Kern A, Rittner K, Kleinschmidt JA. Novel tools for production and purification of recombinant adenoassociated virus vectors. Hum Gene Ther. 1998;9(18):2745-2760.
- Tang Q, Keeler AM, Zhang S, et al. Two-Plasmid Packaging System for Recombinant Adeno-Associated Virus. Biores Open Access. 2020;9(1):219-228. Published 2020 Oct 16.
- Su W, Patrício MI, Duffy MR, Krakowiak JM, Seymour LW, Cawood R. Self-attenuating adenovirus enables production of recombinant adeno-associated virus for high manufacturing yield without contamination. Nat Commun. 2022;13(1):1182. Published 2022 Mar 7.
- Pham DH, Moretti PA, Goodall GJ, Pitson SM. Attenuation of leakiness in doxycycline-inducible expression via incorporation of 3’ AU-rich mRNA destabilizing elements. Biotechniques. 2008;45(2):155-156, 158, 160.
- Karbowniczek K, Extance J, Milsom S, et al. Doggybone™ DNA: an advanced platform for AAV production. Cell Gene Ther Insights. 2017;3(9): 731-738.
- Luke J, Carnes AE, Hodgson CP, Williams JA. Improved antibiotic-free DNA vaccine vectors utilizing a novel RNA based plasmid selection system. Vaccine. 2009;27(46):6454-6459.
- Nyamay’antu A, Hellal M, Porte M, Erbacher P. FectoVIR®-AAV: A giant step for AAV large scale manufacturing. Cell Gene Ther Insights. 2020; 6(4): 655-661.
- Urabe M, Ding C, Kotin RM. Insect cells as a factory to produce adeno-associated virus type 2 vectors. Hum Gene Ther. 2002;13(16):1935-1943.
- Kohlbrenner E, Aslanidi G, Nash K, et al. Successful production of pseudotyped rAAV vectors using a modified baculovirus expression system. Mol Ther. 2005;12(6):1217-1225.
- Smith RH, Levy JR, Kotin RM. A simplified baculovirus-AAV expression vector system coupled with one-step affinity purification yields high-titer rAAV stocks from insect cells. Mol Ther. 2009;17(11):1888-1896.
- Cecchini S, Virag T, Kotin RM. Reproducible high yields of recombinant adeno-associated virus produced using invertebrate cells in 0.02- to 200-liter cultures. Hum Gene Ther. 2011;22(8):1021-1030.
- Chen H. Intron splicing-mediated expression of AAV Rep and Cap genes and production of AAV vectors in insect cells. Mol Ther. 2008;16(5):924-930.
- Chen H. Adeno-associated virus vectors for human gene therapy. World J Med Genet 2015; 5(3): 28-45.
- Galibert L, Jacob A, Savy A, et al. Monobac System-A Single Baculovirus for the Production of rAAV. Microorganisms. 2021;9(9):1799. Published 2021 Aug 24.
- Aslanidi G, Lamb K, Zolotukhin S. An inducible system for highly efficient production of recombinant adeno-associated virus (rAAV) vectors in insect Sf9 cells. Proc Natl Acad Sci U S A. 2009;106(13):5059-5064.
- Mietzsch M, Casteleyn V, Weger S, Zolotukhin S, Heilbronn R. OneBac 2.0: Sf9 Cell Lines for Production of AAV5 Vectors with Enhanced Infectivity and Minimal Encapsidation of Foreign DNA. Hum Gene Ther. 2015;26(10):688-697.
- Mietzsch M, Grasse S, Zurawski C, et al. OneBac: platform for scalable and high-titer production of adeno-associated virus serotype 1-12 vectors for gene therapy. Hum Gene Ther. 2014;25(3):212-222.
- Kondratov O, Marsic D, Crosson SM, et al. Direct Head-to-Head Evaluation of Recombinant Adeno-associated Viral Vectors Manufactured in Human versus Insect Cells. Mol Ther. 2017;25(12):2661-2675.
- Kurasawa JH, Park A, Sowers CR, et al. Chemically Defined, High-Density Insect Cell-Based Expression System for Scalable AAV Vector Production. Mol Ther Methods Clin Dev. 2020;19:330-340. Published 2020 Oct 4.
- Joshi PRH, Venereo-Sanchez A, Chahal PS, Kamen AA. Advancements in molecular design and bioprocessing of recombinant adeno-associated virus gene delivery vectors using the insect-cell baculovirus expression platform. Biotechnol J. 2021;16(4):e2000021.
- Rumachik NG, Malaker SA, Poweleit N, et al. Methods Matter: Standard Production Platforms for Recombinant AAV Produce Chemically and Functionally Distinct Vectors. Mol Ther Methods Clin Dev. 2020;18:98-118. Published 2020 May 22.
- Jorio H, Tran R, Kamen A. Stability of serum-free and purified baculovirus stocks under various storage conditions. Biotechnol Prog. 2006;22(1):319-325.
- Clark KR, Voulgaropoulou F, Fraley DM, Johnson PR. Cell lines for the production of recombinant adeno-associated virus. Hum Gene Ther. 1995;6(10):1329-1341.
- Chadeuf G, Favre D, Tessier J, et al. Efficient recombinant adeno-associated virus production by a stable rep-cap HeLa cell line correlates with adenovirus-induced amplification of the integrated rep-cap genome. J Gene Med. 2000;2(4):260-268.
- Gao GP, Qu G, Faust LZ, et al. High-titer adeno-associated viral vectors from a Rep/Cap cell line and hybrid shuttle virus. Hum Gene Ther. 1998;9(16):2353-2362.
- Mathews LC, Gray JT, Gallagher MR, Snyder RO. Recombinant adeno-associated viral vector production using stable packaging and producer cell lines. Methods Enzymol. 2002;346:393-413.
- Gao GP, Lu F, Sanmiguel JC, et al. Rep/Cap gene amplification and high-yield production of AAV in an A549 cell line expressing Rep/Cap. Mol Ther. 2002;5(5 Pt 1):644-649.
- Giard DJ, Aaronson SA, Todaro GJ, et al. In vitro cultivation of human tumors: establishment of cell lines derived from a series of solid tumors. J Natl Cancer Inst. 1973;51(5):1417-1423.
- Liu XL, Clark KR, Johnson PR. Production of recombinant adeno-associated virus vectors using a packaging cell line and a hybrid recombinant adenovirus. Gene Ther. 1999;6(2):293-299.
- Thomas DL, Wang L, Niamke J, et al. Scalable recombinant adeno-associated virus production using recombinant herpes simplex virus type 1 coinfection of suspension-adapted mammalian cells. Hum Gene Ther. 2009;20(8):861-870.
- Flotte T, Carter B, Conrad C, et al. A phase I study of an adeno-associated virus-CFTR gene vector in adult CF patients with mild lung disease. Hum Gene Ther. 1996;7(9):1145-1159.
- Farson D, Harding TC, Tao L, et al. Development and characterization of a cell line for large-scale, serum-free production of recombinant adeno-associated viral vectors. J Gene Med. 2004;6(12):1369-1381.
- Ogston P, Raj K, Beard P. Productive replication of adeno-associated virus can occur in human papillomavirus type 16 (HPV-16) episome-containing keratinocytes and is augmented by the HPV-16 E2 protein. J Virol. 2000;74(8):3494-3504.
- Taylor TJ, Brockman MA, McNamee EE, Knipe DM. Herpes simplex virus. Front Biosci. 2002;7:d752-d764. Published 2002 Mar 1.
- Bauer DW, Huffman JB, Homa FL, Evilevitch A. Herpes virus genome, the pressure is on. J Am Chem Soc. 2013;135(30):11216-11221.
- Heilbronn R, Engstler M, Weger S, Krahn A, Schetter C, Boshart M. ssDNA-dependent colocalization of adeno-associated virus Rep and herpes simplex virus ICP8 in nuclear replication domains. Nucleic Acids Res. 2003;31(21):6206-6213.
- Weindler FW, Heilbronn R. A subset of herpes simplex virus replication genes provides helper functions for productive adeno-associated virus replication. J Virol. 1991;65(5):2476-2483.
- Alazard-Dany N, Nicolas A, Ploquin A, et al. Definition of herpes simplex virus type 1 helper activities for adeno-associated virus early replication events. PLoS Pathog. 2009;5(3):e1000340.
- Slanina H, Weger S, Stow ND, Kuhrs A, Heilbronn R. Role of the herpes simplex virus helicase-primase complex during adeno-associated virus DNA replication. J Virol. 2006;80(11):5241-5250.
- Meier AF, Tobler K, Leisi R, Lkharrazi A, Ros C, Fraefel C. Herpes simplex virus co-infection facilitates rolling circle replication of the adeno-associated virus genome. PLoS Pathog. 2021;17(6):e1009638. Published 2021 Jun 1.
- Conway JE, Zolotukhin S, Muzyczka N, Hayward GS, Byrne BJ. Recombinant adeno-associated virus type 2 replication and packaging is entirely supported by a herpes simplex virus type 1 amplicon expressing Rep and Cap. J Virol. 1997;71(11):8780-8789.
- Conway JE, Rhys CM, Zolotukhin I, et al. High-titer recombinant adeno-associated virus production utilizing a recombinant herpes simplex virus type I vector expressing AAV-2 Rep and Cap. Gene Ther. 1999;6(6):986-993.
- Rice SA, Knipe DM. Genetic evidence for two distinct transactivation functions of the herpes simplex virus alpha protein ICP27. J Virol. 1990;64(4):1704-1715.
- Hwang KK, Mandell T, Kintner H, Zolotukhin S, Snyder, R, Byrne BJ. High titer recombinant adeno-associated virus production using replication deficient herpes simplex viruses type 1. Mol. Ther. 7, Issue 5. (2003): S14-S15. Accessed at https://www.cell.com/molecular-therapy-family/molecular-therapy/pdf/S1525-0016(16)40477-6.pdf.
- Hakim CH, Clément N, Wasala LP, et al. Micro-dystrophin AAV Vectors Made by Transient Transfection and Herpesvirus System Are Equally Potent in Treating mdx Mouse Muscle Disease. Mol Ther Methods Clin Dev. 2020;18:664-678. Published 2020 Jul 9.
- Trivedi PD, Yu C, Chaudhuri P, et al. Comparison of highly pure rAAV9 vector stocks produced in suspension by PEI transfection or HSV infection reveals striking quantitative and qualitative differences. Mol Ther Methods Clin Dev. 2021;24:154-170. Published 2021 Dec 25.
- Selvaraj N, Wang CK, Bowser B, et al. Detailed Protocol for the Novel and Scalable Viral Vector Upstream Process for AAV Gene Therapy Manufacturing. Hum Gene Ther. 2021;32(15-16):850-861.
- Chulay JD, Ye GJ, Thomas DL, et al. Preclinical evaluation of a recombinant adeno-associated virus vector expressing human alpha-1 antitrypsin made using a recombinant herpes simplex virus production method. Hum Gene Ther. 2011;22(2):155-165.
- Yang Q, Chen F, Trempe JP. Characterization of cell lines that inducibly express the adeno-associated virus Rep proteins. J Virol. 1994;68(8):4847-4856.
- Qiao C, Wang B, Zhu X, Li J, Xiao X. A novel gene expression control system and its use in stable, high-titer 293 cell-based adeno-associated virus packaging cell lines. J Virol. 2002;76(24):13015-13027.
- Tan E, Chin CSH, Lim ZFS, Ng SK. HEK293 Cell Line as a Platform to Produce Recombinant Proteins and Viral Vectors. Front Bioeng Biotechnol. 2021;9:796991. Published 2021 Dec 13.
- Gao K, Li M, Zhong L, et al. Empty Virions In AAV8 Vector Preparations Reduce Transduction Efficiency And May Cause Total Viral Particle Dose-Limiting Side-Effects. Mol Ther Methods Clin Dev. 2014;1(9):20139.
- Pei X, Earley LF, He Y, et al. Efficient Capsid Antigen Presentation From Adeno-Associated Virus Empty Virions In Vivo [published correction appears in Front Immunol. 2020 Feb 04;10:3076]. Front Immunol. 2018;9:844. Published 2018 Apr 19.
- Dash S, Sharon DM, Mullick A, Kamen AA. Only a small fraction of cells produce assembled capsids during transfection-based manufacturing of adeno-associated virus vectors [published online ahead of print, 2022 Feb 19]. Biotechnol Bioeng. 2022;10.1002/ bit.28068.
- Nguyen TNT, Sha S, Hong MS, et al. Mechanistic model for production of recombinant adeno-associated virus via triple transfection of HEK293 cells. Mol Ther Methods Clin Dev. 2021;21:642-655. Published 2021 Apr 16.
- Emmerling VV, Pegel A, Milian EG, et al. Rational plasmid design and bioprocess optimization to enhance recombinant adeno-associated virus (AAV) productivity in mammalian cells. Biotechnol J. 2016;11(2):290-297.
- Mietzsch M, Eddington C, Jose A, et al. Improved Genome Packaging Efficiency of Adeno-associated Virus Vectors Using Rep Hybrids. J Virol. 2021;95(19):e0077321.
- Nicolas A, Jolinon N, Alazard-Dany N, et al. Factors influencing helper-independent adeno-associated virus replication. Virology. 2012;432(1):1-9.
- Satkunanathan S, Wheeler J, Thorpe R, Zhao Y. Establishment of a novel cell line for the enhanced production of recombinant adeno-associated virus vectors for gene therapy. Hum Gene Ther. 2014;25(11):929-941.
- Strasser L, Boi S, Guapo F, et al. Proteomic Landscape of Adeno-Associated Virus (AAV)- Producing HEK293 Cells. Int J Mol Sci. 2021;22(21):11499. Published 2021 Oct 25.
- Barnes CR, Lee H, Ojala DS, Lewis KK, Limsirichai P, Schaffer DV. Genome-wide activation screens to increase adeno-associated virus production. Mol Ther Nucleic Acids. 2021;26:94- 103. Published 2021 Jul 16.
- Grieger JC, Soltys SM, Samulski RJ. Production of Recombinant Adeno-associated Virus Vectors Using Suspension HEK293 Cells and Continuous Harvest of Vector From the Culture Media for GMP FIX and FLT1 Clinical Vector. Mol Ther. 2016;24(2):287-297.
- Adamson-Small L, Potter M, Byrne BJ, Clément N. Sodium Chloride Enhances Recombinant Adeno-Associated Virus Production in a Serum-Free Suspension Manufacturing Platform Using the Herpes Simplex Virus System. Hum Gene Ther Methods. 2017;28(1):1-14.
- Yu C, Trivedi PD, Chaudhuri P, et al. NaCl and KCl mediate log increase in AAV vector particles and infectious titers in a specific/timely manner with the HSV platform. Mol Ther Methods Clin Dev. 2021;21:1-13. Published 2021 Feb 24.
- Li F, Vijayasankaran N, Shen AY, Kiss R, Amanullah A. Cell culture processes for monoclonal antibody production. MAbs. 2010;2(5):466-479.
- Balakrishnan B, Jayandharan GR. Basic biology of adeno-associated virus (AAV) vectors used in gene therapy. Curr Gene Ther. 2014;14(2):86-100. 108. Li C, Samulski RJ. Engineering adeno-associated virus vectors for gene therapy. Nat Rev Genet. 2020;21(4):255-272.
- Li C, Samulski RJ. Engineering adeno-associated virus vectors for gene therapy. Nat Rev Genet. 2020;21(4):255-272.
Subscribe to our e-Newsletters
Stay up to date with the latest news, articles, and events. Plus, get special
offers from American Pharmaceutical Review delivered to your inbox!
Sign up now!