Introduction
Pre-filled syringes (PFS) are well-established, functional container closure systems for biological products. Examples for marketed biological products available as PFS presentation include monoclonal antibody-based products, cytokines and vaccines [1]. PFS do offer some advantages over vials, e.g. (1) ease of use (and thus opportunity for self-administration or administration without further manipulations of the primary container), (2) reduction of medication errors, (3) product differentiation, (4) less overfill and (5) possibility to “combine” with an autoinjector (in case of staked-in needle PFS). The article focuses on the challenges in formulation development which apply specifically to PFS.
Main challenges for a formulation scientist are related to:
- syringeability and
- compatibility
Syringeability
Time and force required for a manual injection (or time required for an injection using an autoinjector) are important and may impact the usability of the product by the end-user and thus compliance. The force required for the injection of a solution at a given injection rate via a needle of predetermined gauge and length is referred to as ‘syringeability’ [2]. The Hagen-Poiseuille equation can be utilized to estimate the travel (or glide) force (Equation 1).
Q = Volumetric flow rate
μ = Fluid viscosity
L = Needle length
R = Needle inner diameter
A = Cross sectional area of syringe plunger
F = Frictionless travel force
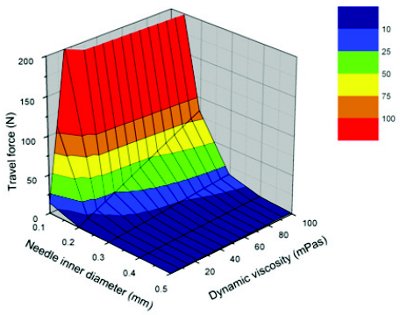
Figure 1 - Calculated travel force as a function of needle inner diameter and dynamic viscosity for an injection rate of 0.1 mL/s using a syringe with an inner diameter of 4.6 mm and a needle of 20 mm length applying the Hagen-Poiseuille equation [3].
According to equation 1, the travel (or glide) force is dependent on a number of parameters. The only parameter a formulation scientist can infl uence is viscosity. All other parameters (needle inner diameter, needle length and cross sectional area of syringe plunger) are determined by the pre-fi llable syringe itself. Figure 1 visualizes the impact of the needle inner diameter and the viscosity on the travel force.
Formulations with a high viscosity can lead to high injection forces and long injection times since both parameters are proportional to viscosity. Generally accepted limits for injection force and injection time do not exist and may depend e.g. on the indication and the dexterity of the patient population. When developing a PFS for an autoinjector, the limit for the injection force is determined by the autoinjector technology. Thus, the technology applied needs to be taken into account for the overall design of the drug product-device combination product. The dimensional attributes of the pre-fi llable syringe need to be adjusted to the viscosity of the formulation and a close collaboration between pharmaceutical scientists and device engineers is mandatory when developing PFS and autoinjector presentations.
Viscosity
The viscosity of a solution is dependent on the protein itself, the protein concentration, the temperature and the formulation, e.g. pH, type of excipients and excipient concentrations.
The viscosities of different monoclonal antibodies at a given concentration can vary signifi cantly even with minor diff erences in the amino acid sequence [4]. In case the target product profi le foresees a high concentration liquid formulation for subcutaneous administration (e.g. a monoclonal antibody in an infl ammation indication), it is prudent to include viscosity as a criterion already during drug candidate selection/molecule assessment to identify early on potential issues for pharmaceutical development.
Besides the protein itself, protein concentration is another important factor for viscosity. Subcutaneous injections are generally limited to an injection volume of approx. < 1.5 mL [5]. Depending on the absolute dose, high concentration liquid formulations may be required to avoid multiple injections. In fact, most monoclonal antibody-based products for subcutaneous administration on the market are high protein concentration formulations, due to absolute doses often in the mg/kg range [5]. In some cases, multiple injections or larger s.c. injection volumes may be required to achieve the desired clinical dose, given that the achievable protein concentration is physically limited. High protein concentration formulations of biologics have viscosities typically much higher than the viscosity of water. The solution behavior may signifi cantly deviate from the ideal solution behavior due to protein-protein interactions which could cause an increase in viscosity. For monoclonal antibodies, the viscosity usually increases exponentially at a concentration of > 50 to 100 mg/mL [2, 4, 6, 7]. During formulation development of a new biological entity, the fi nal dose it usually not known. In this case, a protein concentration “as high as technically feasible” is usually aimed for when developing PFS presentations. The impact of the protein concentration on product stability [8] needs to be assessed as well as the impact of viscosity on the drug substance (DS) and the drug product (DP) manufacturing process [5, 7], and on syringeability [2]. The formulation scientist needs to consider not only the viscosity at the nominal protein concentration but also the viscosity at the upper specifi cation limit (e.g. nominal protein concentration + 10%) and the protein concentration during DS manufacturing which can be signifi cantly higher.
Most biological products are stored at 2 to 8°C. To avoid injection pain, the PFS (or autoinjector) is typically equilibrated to room temperature prior injection. However, the eff ect of temperature on viscosity needs to be investigated, e.g. to assess the impact of lacking or incomplete temperature equilibration on syringeability which could result in longer injection times or even functionality failure of the PFS and/or autoinjector and thus leading to variations in injection time or even failure in delivering the intended dose.
Once a molecule and the target concentration have been chosen, the formulation scientist can only infl uence the viscosity by the formulation parameters, e.g. pH, ionic strength and type of excipient can have a signifi cant impact on viscosity [7, 9]. However, the approaches to reduce viscosity by adjusting pH and/or ionic strength are typically limited, as these parameters infl uence the stability of the protein as well. Especially chemical degradation (e.g. deamidation or succinimide formation) is pH dependent [10]. Thus, the optimum formulation parameters for viscosity and stability can be quite diff erent [7] and need to be balanced.
Once a formulation has been chosen, injection force and injection time can only be reduced for a defi ned syringe size by increasing the needle inner diameter and to a lesser extent by decreasing the needle length. However, increasing the needle size has its limitations as it may potentially impact pain perception leading to a competitive disadvantage. Thin wall needles may be an option to increase the needle inner diameter of a cannula while maintaining the same needle outer diameter.
Compatibility
The number of compounds which may leach into the DP solution is –in sheer number- much higher for PFS compared to vial presentations as the number of components which are part of the container closure system is higher. Besides an assessment of (potentially) leaching components on patient safety, the potential to induce or accelerate protein degradation needs to be considered during formulation development.
Tungsten
Tungsten pins are routinely used by manufacturers of glass pre-fillable syringes in the syringe manufacturing process to keep the bore open while the cone is being shaped. The shaping process requires application of high temperatures. Although tungsten is quite resistant, metal particles may be deposited from the pins during the process. In addition, oxidation processes occur leading to formation of tungsten oxides that may subsequently deposit in the bore and funnel area of the syringe. In this respect it is important to keep in mind that deposits of tungsten species can occur in staked-in needle as well as luer type pre-fillable syringes (although staked-in needle pre-fillable syringes contain the lowest amount of extractable tungsten as most of the bore is covered by the needle [11]).
When exposed to an aqueous solution with an acidic pH of about 6 or below, both tungsten metal and tungsten oxide can form a variety of soluble polyanionic species which can induce protein aggregation and formation of proteinaceous particles [12-15]. Protein oxidation was reported to be induced by tungsten as well leading to protein aggregation [16, 17]. Other formulation parameters which have been reported to have an influence on tungsten induced protein degradation besides pH are protein concentration and ionic strength [18]. Thus, pH, protein concentration and ionic strength of a formulation should be taken into account in the risk assessment for tungsten compatibility of a formulation.
The syringe-to-syringe variability of residual tungsten can be significant. Stability testing in the actual PFS can to a certain degree assess also compatibility with tungsten, however, with the caveat of variability of these deposits. Other approaches to assess tungsten compatibility are e.g. spiking with soluble tungsten species (e.g. Na2WO4), spiking with an extract from used tungsten pins or spiking with an extract from the glass barrel. It should be mentioned that depending on the approach chosen, results can significantly differ. Spiking with an extract of a used tungsten pins induced protein degradation more effectively than spiking with soluble tungsten species including WO3, H2WO4 and Na2WO4 in an example by Jiang et al. [14].
Overall, the number of reported tungsten incompatibilities in biological products at tungsten concentrations relevant for PFS is low, given the widespread use of PFS in the field of biologics.
Nevertheless, pre-fillable syringes with low(er) amounts of residual tungsten levels are meanwhile available by different syringe vendors. Some suppliers also tried replacing tungsten pins by other materials, such as transition metals. However, also leachables from these components cannot be ruled out to generate significant and potentially more severe issues than tungsten.
Silicone Oil
Silicone oil or silicone oil emulsion is used to lubricate the inner wall of the glass barrels of pre-fillable syringes to ensure functionality of the PFS and/or autoinjector over its shelf life.
Silicone oil has been reported to induce protein aggregation of some model proteins in non-optimized formulations under quiescent storage at 45°C [19] and to increase protein aggregation upon agitation stress [20]. However, the spiked silicone oil concentrations were much higher than typically observed in PFS. In any case, agitation stress studies of candidate formulations in PFS should be part of formulation screens in addition to quiescent stability studies.
Abatacept, a Fc fusion protein on the market as lyophilized formulation, is one of the few biological products with a reported silicone oil incompatibility upon quiescent storage. However, abatacept has been reported to form visible particles upon storage at 25°C in solution even in non-siliconized syringes although formation of visible particles was much faster in case of siliconized pre-fillable syringes [21]. Therefore, silicone-free disposable syringes are supplied with this drug and are recommended to be used for reconstitution and dilution.
To assess silicone oil compatibility, stability can be tested in containers with worst case silicone oil amount, e.g. Auge et al. [22] tested the compatibility in containers up to 6x the target silicone level. An alternative are silicone oil spiking studies [19, 20, 23].
A minimum silicone oil quantity is necessary to ensure functionality over shelf life of the product. Therefore reducing the silicone quantity per syringe to counteract silicone oil incompatibility may not be feasible or even a concern. Silicone-free plastic syringes may also not provide a suitable option as these systems are still not well established yet and are associated with other challenges (e.g. oxygen permeability, potential leachables and surface sensitivity).
Another challenge associated with silicone oil is related to the formation of silicone oil droplets in the sub-visible particle size range. Once the silicone oil detaches from the inner wall of the glass barrel, silicone oil droplets are formed and contribute to the sub-visible particle population. Silicone oil droplets can be found in PFS independent of the siliconization process, i.e. in PFS siliconized by spray-on as well as baked siliconization [24]. Thus, the sub-visible particle population in PFS is more complex compared to vials and consists of extraneous particulate matter, silicone oil droplets and potentially proteinaceous sub-visible particles. Formation of proteinaceous sub-visible particles is a potential degradation pathway of biologics [25] and has therefore gained increased attention by health authorities [12, 26]. The concern was raised that proteinaceous sub-visible particles may potentially induce immunogenicity. Thus, it becomes very important to characterize the sub-visible particle population and to be able to discriminate between silicone oil droplets and proteinaceous sub-visible particles. Light obscuration is the standard pharmacopoeial method to quantify sub-visible particles. However, the method is not able to differentiate types of particles. Flow microscopy is currently one of the available methods to discriminate between types of particles [27]. Most flow microscopy systems optimized for particle detection capture bright field images as a test solution flows through a narrow rectangular channel under stroboscopic illumination [28]. The images are analyzed by the system software and provide information on the size and concentration of the particles as well as the shape/morphology (e.g. aspect ratio or circularity). The shape/morphology information can help to discriminate between types of particles. So-called ‘silicone oil software filters’ can be helpful to discriminate between silicone oil droplets and proteinaceous sub-visible particles. However, the error using a simple silicone oil software filter solely based on size and aspect ratio as previously described in literature [29] can be quite considerable leading to a significant fraction of proteinaceous sub-visible particles misclassified as silicone oil droplets [30, 31]. Thus, more sophisticated silicone oil software filters should be developed which are able to reduce misclassification error [31]. However, it is questionable whether a reliable differentiation of sub-visible particles < 4μm with flow microscopy is possible at all due to limitations of the optical system (limitations due to diffraction and wavelength) and the sensor (limitations due to sampling constraints and the size of the actual photo site that can be produced on the sensor) [32]. One of the emerging methods is the so-called ‘Archimedes nanomechanical resonator system’, which might be able to discriminate between silicone oil droplets and proteinaceous sub-visible particles below the size limit of flow microscopy instruments down to about 500 nm. It relies on small, momentary changes in resonant frequency caused by passage of particles through a micro-channel within a cantilever. The magnitude of the frequency change is proportional to the size and density of the particle [33].
Although there is certainly the need to differentiate types of particles in the sub-visible and in the submicron particle size range, there is currently no method available which covers the full size range and is mature enough to be used on a routine basis.
Adhesives
Adhesives are utilized to attach the needle in staked-in needle pre-fillable syringes. The adhesive is usually cured by UV light. An incomplete UV curing process led to an unexpected impurity found in a new staked-in needle PFS presentation for a biological product which has not been previously observed in a luer cone PFS presentation [30]. Process improvements by the syringe vendor could dramatically decrease the impurity levels in the syringe. The impact on the DP stability was tested but has not been observed.
In another reported case, the solvent from a partially dried adhesive leached into the DP solution. The leached solvent led to an increased protein oxidation [34].
Needle Shield Rubber Components
Thyagarajapuram [35] identified the root cause of clogged needles as protein aggregation which led to a gel-like clog. Induced protein aggregation was related to leachables from certain types of needle shields while others did not have any effect. Zinc plays a role but does not seem to be the only reason. Another observation made was a decreased polysorbate concentration which could indirectly lead to instabilities.
Concluding Remarks
Developing a PFS presentation for a biological entity is much more complex compared to developing a vial configuration as the container closure system consists of more components and as functionality over the product shelf life needs to be ensured. Therefore it is important to test the stability of candidate formulations in PFS before selecting the final formulation. In case a new biological entity is to be launched as a PFS presentation, it is typically being tested in clinical studies such as the pivotal clinical studies. Thus, formulation and PFS development needs to start at a point in time when the required therapeutic dose is not known which adds further challenges.
Further research and development is necessary to:
- reliably predict the viscosity based on primary structure and homology modeling using in silico methods
- increase the choice of viscosity reducing excipients
- extend autoinjector operating range with regards to viscosity
- extend DS and DP manufacturing process operating ranges with regards to viscosity
- explore the maximum tolerable subcutaneous injection volume
- better predict human in vivo injection times based on in vitro models
- better understand the impact of protein and formulation parameters on tungsten and silicone oil compatibility
- improve capabilities of analytical methodologies for the characterization of sub-visible and submicron particles in terms of size limitations and in terms of particle type discrimination
Acknowledgements
The author would like to gratefully thank Robert Müller, Pierre Goldbach and Hanns-Christian Mahler for support in the preparation of the manuscript.
References
- Walsh, G. Pharmaceutical biotechnology products approved within the European Union. Eur. J. Pharm. Biopharm. 2003, 55 (1), 3-10.
- Burckbuchler, V.; Mekhloufi, G.; Paillard Giteau, A.; Grossiord, J.L.; Huille, S.; Agnely, F. Rheological and Syringeability Properties of Highly Concentrated Human Polyclonal Immunoglobulin Solutions. Eur. J. Pharm. Biopharm. 2010, 76 (3), 351-356.
- Müller, R. Characterization of a High Concentration Antibody Formulation: Protein Stability and Formulation Properties. Ph.D. Thesis, University of Halle-Wittenberg, Germany, 2011.
- Yadav, S.; Alavattam, S.; Kanai, S.; Liu, J.; Lien, S.; Lowman, H.; Kalonia, D.S.; Shire, S.J. Establishing a Link Between Amino Acid Sequences and Self-Associating and Viscoelastic Behavior of Two Closely Related Monoclonal Antibodies. Pharm. Res. 2011, 28 (7), 1750-1764.
- Shire, S.J.; Shahrokh, Z.; Liu, J. Challenges in the Development of High Protein Concentration Formulations. J. Pharm. Sci. 2004, 93 (6), 1390-1402.
- Kanai, S.; Liu, J.; Patapoff, T.W.; Shire, S.J. Reversible Self-association of a Concentrated Monoclonal Antibody Solution Mediated by Fab-Fab Interaction that Impacts Solution Viscosity. J. Pharm. Sci. 2008, 97 (10), 4219-4227.
- Shire, S.J. Formulation and Manufacturability of Biologics. Curr. Opin. Biotechnol. 2009, 20 (6), 708-714.
- Treuheit, M.J.; Kosky, A.A.; Brems, D.N. Inverse Relationship of Protein Concentration and Aggregation. Pharm. Res. 2002, 19 (4), 511-516.
- Liu, J.; Nguyen, M.D.H.; Andya, J.D.; Shire, S.J. Reversible Self-Association Increases the Viscosity of a Concentrated Monoclonal Antibody in Aqueous Solution. J. Pharm. Sci. 2005, 94 (9), 1928-1940.
- Manning, M.C.; Chou, D.K.; Murphy, B.M.; Payne, R.W.; Katayama, D.S. Stability of protein pharmaceuticals: an update. Pharm. Res. 2010, 27 (4), 544-575.
- Fries, A. Drug Delivery of Sensitive Biopharmaceuticals with Prefilled Syringes. Drug Delivery Technol. 2009, 9 (5), 22-26.
- Rosenberg, A.S. Effects of Protein Aggregates: An Immunologic Perspective. AAPS J. 2006, 8 (3), E501-E507.
- Bee, J.S.; Nelson, S.A.; Freund, E.; Carpenter, J.F.; Randolph, T.W. Precipitation of a Monoclonal Antibody by Soluble Tungsten. J. Pharm. Sci. 2009, 98 (9), 3290-3301.
- Jiang, Y.; Nashed-Samuel, Y.; Li, C.; Liu, W.; Pollastrini, J.; Mallard, D.; Wen, Z.-Q.; Fujimori, K.; Pallitto, M.; Donahue, L.; Chu, G.; Torraca, G.; Vance, A.; Mire-Sluis, A.; Freund, E.; Davis, J.; Narhi, L. Tungsten-Induced Protein Aggregation: Solution Behavior. J. Pharm. Sci. 2009, 98 (12), 4695-4710.
- Liu, W.; Swift, R.; Torraca, G.; Nashed-Samuel, Y.; Wen, Z.-Q.; Jiang, Y.; Vance, A.; Mire-Sluis, A.; Freund, E.; Davis, J.; Narhi, L. Root Cause Analysis of Tungsten-Induced Protein Aggregation in Pre-filled Syringes. PDA J. Pharm. Sci. Technol. 2010, 64 (1), 11-19.
- Osterberg, R.E. Potential Toxicity of Extractables and Leachables in Drug Products. Am. Pharm. Rev. 2005, 8 (2), 64-67.
- Markovic, I. Challenges Associated with Extractable and/or Leachable Substances in Therapeutic Biologic Protein Products. Am. Pharm. Rev. 2006, 9 (6): 20-27.
- Waxman, L.; Steeley, T.; Vilivalam, V. Development of an Assay to Determine Protein Sensitivity to Tungsten and to Investigate the Effects of pH and Ionic Strength on Aggregation. www.westpharma.com/en/support/Pages/TechnicalResources.aspx ., accessed November 22, 2011.
- Jones, L.S.; Kaufmann, A.; Middaugh, C.R. Silicone Oil Induced Aggregation of Proteins. J. Pharm. Sci. 2005, 94 (4), 918-927.
- Thirumangalathu, R.; Krishnan, S.; Speed Ricci, M.; Brems, D.N.; Randolph, T.W.; Carpenter, J.F. Silicone Oil- and Agitation-Induced Aggregation of a Monoclonal Antibody in Aqueous Solution. J. Pharm. Sci. 2009, 98 (9), 3167-3181.
- Majumdar, S.; Ford, B.M.; Mar, K.D.; Sullivan, V.J.; Ulrich, R.G.; D’Souza, A.J.M. Evaluation of the Effect of Syringe Surfaces on Protein Formulations. J. Pharm. Sci. 2011, 100 (7), 2563-2573.
- Auge, K.B.; Blake-Haskins, A.W.; Devine, S.; Rizvi, S.; Li, Y.-M.; Hesselberg, M.; Orvisky, E.; Affleck, A.P.; Spitznagel, T.M.; Perkins, M.D. Demonstrating the Stability of Albinterferon Alfa-2b in the Presence of Silicone Oil. J. Pharm. Sci. 2011, 100 (12), 5100-5114.
- Badkar, A.; Wolf, A.; Bohack, L.; Kolhe, P. Development of Biotechnology Products in Pre-filled Syringes: Technical Considerations and Approaches. AAPS PharmSciTech. 2011, 12 (2), 564-572.
- Adler, M. Detection, Quantification and Identification of Subvisible and Visible Particles in Protein Formulation Development. Presented at the BioProcess International European Conference, Vienna, Austria, May 19-20, 2010.
- Mahler, H.-C.; Friess, W.; Grauschopf, U.; Kiese, S. Protein Aggregation: Pathways, Induction Factors and Analysis. J. Pharm. Sci. 2009, 98 (9), 2909-2934.
- Carpenter, J.F.; Randolph, T.W.; Jiskoot, W.; Crommelin, D.J.A.; Middaugh, C.R.; Winter, G.; Fan, Y.-X.; Kirshner, S.; Verthelyi, D.; Kozlowski, S.; Clouse, K.A.; Swann, P.G.; Rosenberg, A.; Cherney, B. Overlooking Subvisible Particles in Therapeutic Protein Products: Gaps That May Compromise Product Quality. J. Pharm. Sci. 2009, 98 (4), 1201-1205.
- Sharma, D.K.; King, D.; Moore, P.; Oma, P., Thomas, D. Flow Microscopy for Particulate Analysis in Parenteral and Pharmaceutical Fluids. Eur. J. Parent. Pharm. Sci. 2007, 12 (4), 97–101.
- Ripple, D.C.; Wayment, J.R.; Carrier M.J. Standards for the Optical Detection of Protein Particles. Am. Pharm. Rev. 2011, 4, 90-96.
- Sharma, D.K.; Oma, P.; Krishnan, S. Silicone Microdroplets in Protein Formulations. Pharm. Tech. 2009, 33 (4), 74-79.
- Adler, M. Challenges in development of pre-filled syringes for biologics – a formulation scientist‘s point of view. Presented at the 2011 PDA Europe The Universe of Pre-filled Syringes and Injection Devices, Basel, Switzerland, November 7-11, 2011.
- Strehl, R.; Rombach-Riegraf, V.; Diez, M.; Egodage, K.; Bluemel, M.; Jeschke, M.; Koulov, A.V. Discrimination Between Silicone Oil Droplets and Protein Aggregates in Biopharmaceuticals: A Novel Multiparametric Image Filter for Sub-visible Particles in Microflow Imaging Analysis. Pharm. Res. [Online First]. DOI: 10.1007/s11095-011-0590-7. Published Online: September 27, 2011.
- Brown, L. Imaging Particle Analysis: Resolution and Sampling Considerations. www.fluidimaging.com/resource-center-whitepapers.htm , accessed November 22, 2011.
- Patel, A. Identifying and Characterizing Subvisible Particles in the 500 nm – 5 m Range using the Archimedes Nanomechanical Resonator System. Presented at the IBC Well Characterized Biologicals, Washington DC, USA, October 5, 2011.
- Markovic, I. Considerations for Extractables and Leachables in Single Use Systems: A Risk-Based Perspective. Presented at the PDA Single Use Systems Workshop, Bethesda, USA, June 22-23, 2011.
- Thyagarajapuram, N.R. Biopharmaceutics Formulation Development in Pre-filled Syringe as Combination Product. Presented at the 2011 PDA Europe The Universe of Pre-filled Syringes and Injection Devices, Basel, Switzerland, November 7-11, 2011.
Author Biography
Dr. Michael Adler is a pharmacist by training holding a Ph. D. degree in pharmaceutical technology. In 1999, he joined Knoll/BASF Pharma (later Abbott Laboratories) as Lab Head for Parenteral Dosage Forms. In this position, he was involved in formulation and process development activities for biologics and small molecule parenteral products.
In 2006, he joined Hoffmann La-Roche in Formulation R&D Biologics with a focus on early stage formulation development of biologics, especially monoclonal antibodies. In his current position as Head of Late-Stage Pharmaceutical and Processing Development, his department is responsible for market formulation, process development, technology transfer and process validation of both biologics and small molecule parenteral products.