Introduction
Nucleic acid therapeutics have emerged as new class of potential drugs for targeting and treatment of various diseases. In recent years, there has been considerable interest in finding appropriate technologies to formulate nucleic acids into appropriate and bioavailable dosage forms, especially the mRNA.1 As a result of the introduction of life-saving COVID-19 vaccines, and the launch of small interfering RNA (siRNA) as polyneuropathy drug by Alnylam, the industry is renewing interest in the next generation of lipid nanoparticle technology for delivery of mRNA therapeutics for cancers, anti-infl ammatory, and rare diseases, among others. Figure 1 illustrates the innovation in LNP technologies that have resulted in the launch of several small molecules, peptide, nucleic acids, and biologics.3/2
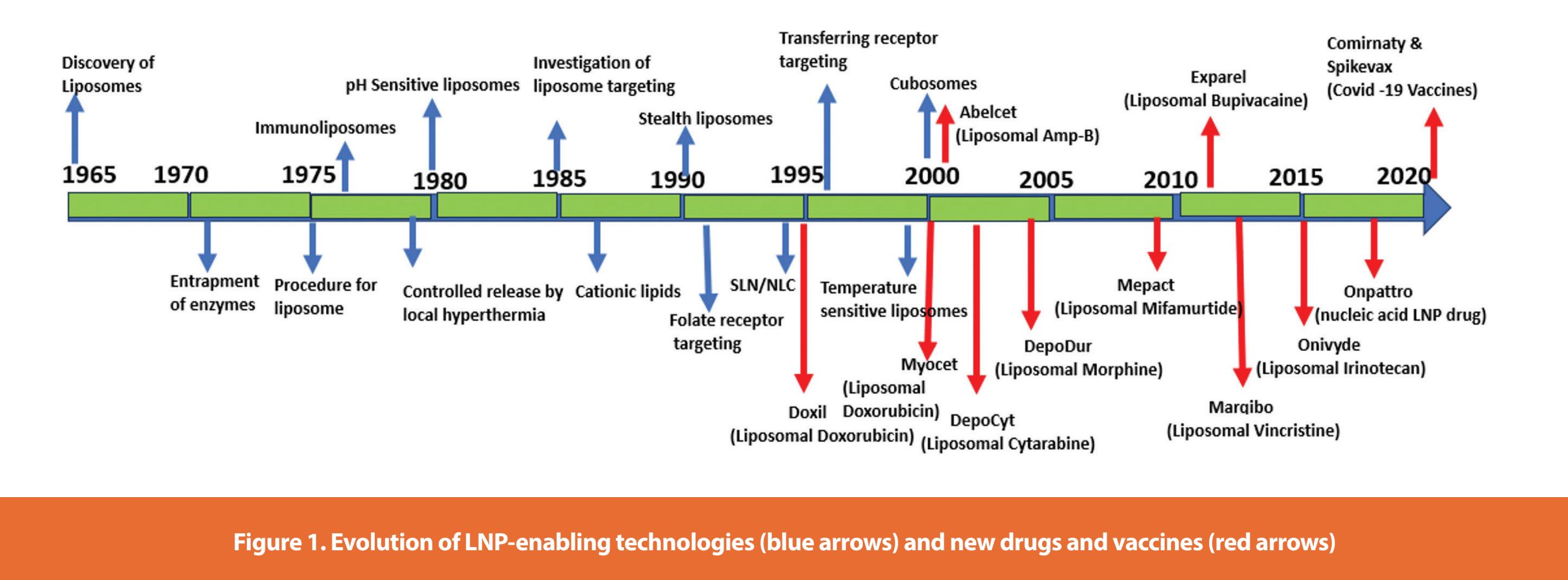
LNP is a complex structure. As a result, the encapsulation and delivery of nucleic acids in efficient and effective manners creates challenges and opportunities. For instance, these lipid assemblies have been proven to be ideal vehicles for intracellular delivery of mRNA4,5,6/3/4/5 due to their inherent abilities to protect the delicate nucleic acids from degradation under aqueous and nuclease environment.7/6 The encapsulation of mRNA into LNPs, however warrants a closer scrutiny since every components of LNPs, especially the cationic lipids, plays a crucial role in protecting, delivery and stability of these large macromolecules.8/7
Structure of LNPs with nucleic acids
LNP used in encapsulation of negatively charged nucleic acids are typically comprised of four components. Most of the ingredients used in the marketed drugs are also listed in FDA inactive ingredient database. It is evident that the negatively charged nucleic acid is complexed with positively charged ionizable lipids (lipoplex) and is entrapped within the LNPs. Designed with helper phospholipids such as di-stearoyl phosphocholine and cholesterol, these lipids are distributed asymmetrically to create an outer stable monolayer boundary, while the pegylated lipids are situated outside for providing steric stability of core surface. As shown in Figure 2, the LNP interior is comprised of electrostatically neutral inverted micelles, in which nucleic acid is surrounded by the ionizable cationic lipid and other lipid components. The surface of the LNP is composed of a hydrophilic shell containing the PEG‐lipid.17/8
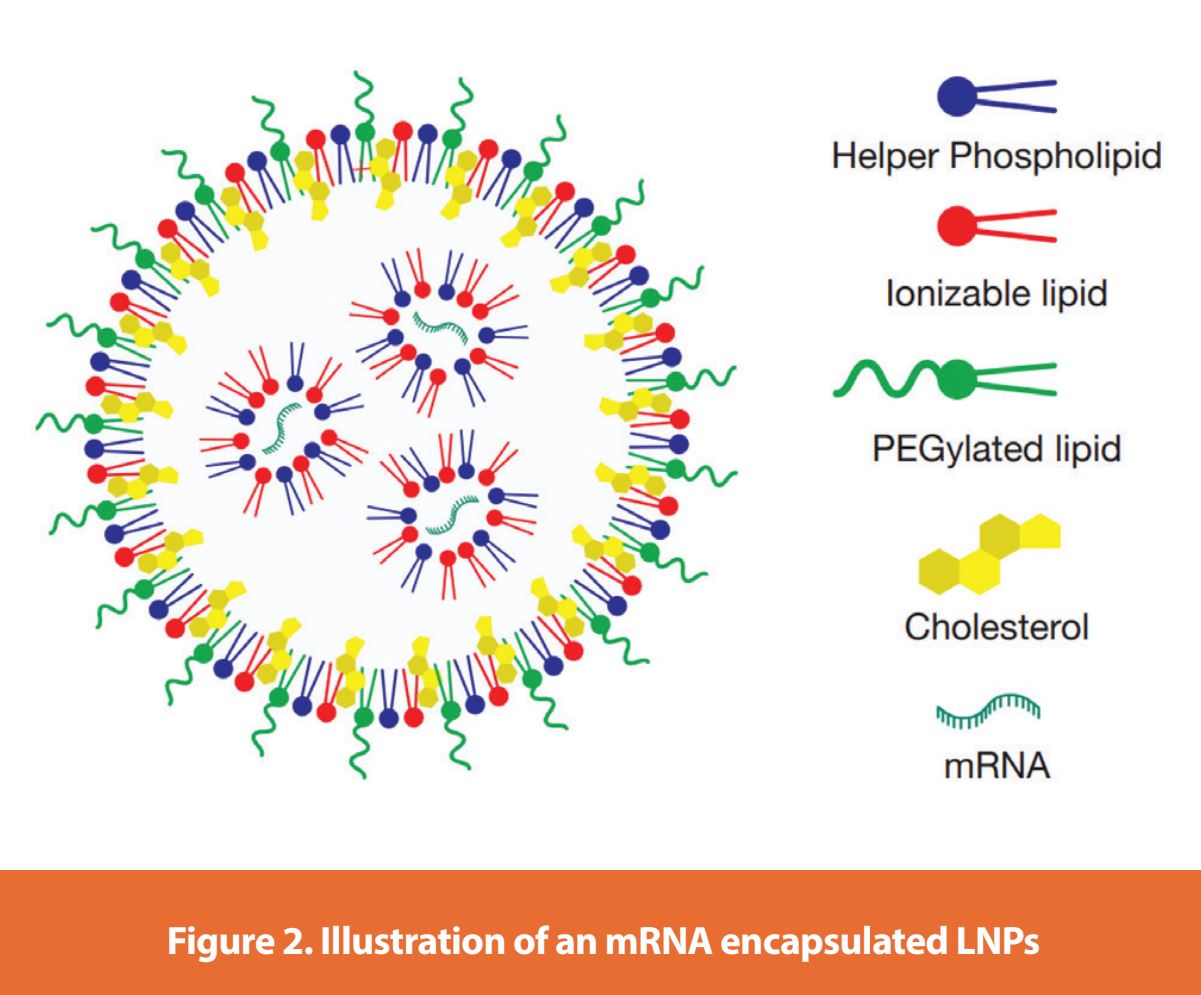
Ionizable lipid nanoparticles (iLNPs) are comprised of cationic lipids with amino moiety as head group, PEGylated lipid, and helper lipids, including phospholipid and cholesterol, which provide the stability of the outer layer core. The amino head group of the ionizable lipids are typically tertiary amine with pKa 6.2-6.9. Those ionizable lipids available commercially are MC3 and ALC-0315 in Comirnaty vaccine (Pfizer/BioNTech) and SM-102 in Spikevax vaccine (Moderna).
Manufacturing of LNPs
These amphiphilic lipids can spontaneously aggregate into LNPs in aqueous solution. The process requires direct mixing of lipid (organic) and nucleic acids (aq. solution) by agitation that results in encapsulation of negatively charged mRNA. Hydrophobic fatty acid chains and polar headgroup help create the lipid assemblies that can further be sized into desired particle sizes. Top-down and bottom-up approaches are common for generating these particles.
Top-down requires high shear and high energy where the lipid (dried fi lm) is homogenized in aqueous buff er to yield desired particle size. In a bottom-up approach, such as nanoprecipitation requires, the ethanol injection that results in formation of nanoparticles. Shepherd et al. (2023) demonstrated the formation of LNPs (comprised of ionic lipid D-Lin-MC3-DMA:DSPC:Cholesterol:DMG-PEG 2000; 50:10:38.5:1.5) in high throughout production with comparable and uniform particle size (ca. 70 nm) with low polydispersity index (ca. 0.004), high encapsulation effi ciency (ca. 88%).9
Scale up manufacturing requires a T- or Y-mixer for rapid mixing of lamellar fl ow liquids into a turbulent fl ow at the mixing point.26/10 By changing the lipid composition, volume or fl ow rate ratio between the two phases (organic/aqueous), the LNP size can be controlled. For typical mRNA encapsulation, one part of lipid solution is mixed with three parts of aqueous phase. Downstream tangential fl ow fi ltration (TFF) is used to concentrate the LNPs, to remove ethanol, any residual lipids and non-encapsulated nucleic acids, and to neutralize the formulation to the target pH of 7.4. The authors demonstrated that for LNPs generated by the continuous T- or Y- mixing modes, those formulations meet the quality critical attributes of mRNA vaccines with particle size of 71 nm and EE of 88%. This continuous process can potentially save time and reduce costs.
Ascendia’s LipidSol® platform technology leverages different process methods to make LNPs carrying various therapeutic modalities with both hydrophilic and lipophilic properties.3 Coupled with lab-scale screening and cGMP sterile manufacturing capabilities, Ascendia is leading the way in design, development, and manufacturing of LNPs for novel therapeutics in cancers, infectious and many other rare diseases.
References
- Nature Editorial, Let’s talk about lipid nanoparticles, Nature Reviews – Materials, 2021, 6, 99.
- R. Tenchov, R. Bird, A. E. Curtze, and Q. Zhou, Lipid Nanoparticles from liposomes to mRNA vaccine delivery, a landscape of research diversity and advancement, ACS Nano, 2021. 15, 16982-17015.
- J. Huang and S. Ali, LipidSol® - a lipid nanoparticle technology for drug delivery, Drug Development & Delivery, 2023.
- S. Guan and J Rosenecker, Nanotechnologies in delivery of mRNA therapeutics using nonviral vector-based delivery systems, Gene Ther., 2017, 24, 133-143.
- A. M. Reichmuth, M. A. Oberli, A. Jaklenec, R. Langer, and D. Blankschtein, mRNA vaccine delivery using lipid nanoparticles. Therapeutic Delivery, 2016, 7, 319–334.
- S. C Semple, A. Akinc, J. Chen, A. P. Sandhu, B. L. Mui, C. K Cho, D. W Y Sah, D. Stebbing, E. J Crosley, E. Yaworski, I. M Hafez, J et al., 2010, Rational design of cationic lipids for siRNA delivery, Nature Biotech., 2010, 28, 172–176.
- C. Gueguen, T. Ben Chimol, M. Briand, K. Renaud, M. Seiler, M. Ziesel, P. Erbacher, and M. Hellal, Evaluating how cationic lipid aff ects mRNA-LNP physical properties and biodistribution, Eur. J. Pharm. Pharmacol., DOI: https://doi.org/10.1016/j.ejpb.2023.08.002
- R. N. Kularatne, R. M. Crist and S. T. Stern, The Future of tissue-targeted lipid nanoparticle- mediated nucleic acid delivery, Pharmaceutics, 2022, 15, 897; doi.org/10.3390/ph15070897
- S. J. Shepherd, X. Han, A. J. Mukalel, R. El-Mayta, A. S. Thatte, J. Wu, M. S. Padilla, M. G. Alameh, N. Sirkumar, D. Lee, D. Weissman, D. Issadore, and M. J. Mitchell, PNAS, 2023, 120, 1-12.
Author Details
Shaukat Ali, PhD and Jim Huang, PhD Ascendia® Pharmaceuticals, Inc.
Publication Detail
This article appeared in American Pharmaceutical Review:
Vol. 27, No. 2
Innovations at Interphex 2024
Pages: 16-17
Subscribe to our e-Newsletters
Stay up to date with the latest news, articles, and events. Plus, get special
offers from American Pharmaceutical Review delivered to your inbox!
Sign up now!