Importance of Stressed Populations for Microbial Testing in Industry
The goal of microbial testing is to detect contamination that is present in, or introduced into sample matrices such as controlled manufacturing environments (air, surfaces, personnel), waters, and raw, in-process, and final product materials among many others. Like any testing application, the methods must be validated to confirm their effectiveness in detecting microbial contaminants from the particular sample matrix in question. All too often such validation work utilizes USP\EP test species, or at best in-house microbial isolates grown in batch culture in rich, non-selective media as the test populations. While useful in the validation process, the use of such challenge preparations does not address any stress conditions that microbial contaminants may experience in such environments. This can result in a “validated” test method that can fail to detect microbial contaminants that may be present in the test sample, and leading to an uncontrolled state in the facility.
Recently, this fact has become apparent to many regulatory bodies and the industry [1, 2]. Thus, use of stressed populations, or samples containing in-situ bioburden is being emphasized for the validation of new microbial testing applications. The use of in-situ microbial samples is easily implemented in cases such as water testing, however in other instances this may not be the feasible due to the low levels of bioburden present. In such situations, artificially stressed populations may need to be prepared and used to model stressed cells that may be present in the samples matrices. The use of such populations can then aid in validating new testing applications whether in EM or such areas as end-product or in-process bioburden testing. It should be noted that the use of stressed populations for the validation of rapid methods is of particular importance as a major goal of rapid methods is to reduce the time to result. A failure to validate a rapid method with stressed test populations could result in the selection of a time to result that will not detect contaminating bioburden.
Real World Microbes are Stressed
The understanding of the growth dynamics of microbial cells was colored by studies that utilized laboratory grown cultures which resulted in the erroneous assumption among many that this is also the normal state of microbes in the environment outside the laboratory culture flask. over the last 20-30 years, a fuller though not complete understanding of the stressed state in microbial populations has been achieved. This work has made it clear that microbes in the natural environment exist in a constant state of stress, and that unstressed batch culture grown microbes are an artificial creation that rarely would exist outside the laboratory.
Real world microbial environments are varied and consist of specific sample matrices or surroundings in which a microbial colony forming unit (CFU) must survive inclusive of physical and chemical characteristics such as temperature, pH, water content and nutrient levels. in pharmaceutical manufacturing settings, environments can range from stainless steel surfaces, tyvek suit material, or latex gloves to purified water loops and fill lines etc. Additionally, raw materials inprocess production streams, final product and associated handling equipment are also potential environments that microbes may suddenly find themselves having to survive in or on. it is likely that at least one of the inherent physical or chemical components present will cause stress to microbial cells in some form as defined by the response of stress response machinery in the cell at both genetic and physiological levels [3, 4].
Table 1 presents examples of particular environments and stresses that may be present for controlled areas, raw materials, and finished product.
These examples demonstrate that in any environment more than one stress factor may be present. Each factor may act synergistically with the others to increase the stress or injury to microbial populations, or to induce protective responses in the populations that will interfere with control procedures. Stress factors also may serve as selective agents leading to the establishment of microbial populations that are able to adapt to the particular environments. For example, microbial populations in water systems are composed almost exclusively of gram-negative species capable of forming biofilms, while air bioburden consists mainly of gram-positive bacteria and mold species [4].
Table 1. Stress factors associated with environments
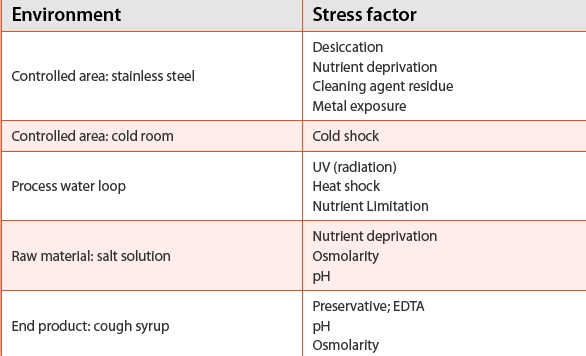
Stress and Injury Effects on Microbial Growth
The growth dynamics of a stressed cell is driven by whether the cell is injured or not. Responses to levels of stress such as a mild temperature up-shift in the culture temperature will result in an extended doubling time of the cell population as the general physiology shifts to meet the new environmental state. once cell resources and physiology are adjusted, the cell doubling time will again resume at the normal rate for the conditions present. However following cell injury, growth pauses as the cell devotes its resources to repair or replace damaged components and systems. For example, heat shock of bacterial cells lead to dNA strand breaks, protein denaturation, and cell envelope disruption. once the damage has been repaired, growth would then resume. Since a stressed population contains a mix of cells with different degrees of injury (or none at all), growth as measured by an increase in cell numbers would exhibit a growth dynamic exhibited by a longer lag phase after injury. Studies that examined early growth of injured populations have shown that significant lag exists before growth in individual cells after injury. This delay demonstrated that injured cells are unable to divide until critical cell machinery is repaired or replaced [6, 7].
Preparation of Stressed Populations for Method Validation
Microbial testing methods are utilized for the detection of microbes captured from real world samples whether from environmental monitoring (air, surfaces, and personnel), raw or in-process materials or final product. laboratory populations grown in batch culture or obtained commercially do not reflect the same state as organisms obtained from test samples. To be confident that a test method is effective in detecting microbial contaminants, it must be validated using microbial populations exposed to the same environmental stresses as are present in the sample. in many cases this is possible as bioburden in situ in many samples is sufficient to allow validation testing to be performed. Bioburden testing in potable water samples is an example where the use of in situ bioburden would be feasible. However in many situations, limited or non-existent microbial counts in the sample matrix prevents such testing (e. g. in WFi loops). in these situations, stressed populations can be prepared to model the effects of the stress factor(s) on the test population growth and recovery, thus providing a more robust assessment of the suitability of the new method.
Model stressed populations should reflect the bioburden of the sample, and mimic exposure to the stress factors that are present in the sample matrix as closely as possible. in order to achieve this, the isolates obtained from the sample environment should be identified and used. Also, stress factors must be defined in order to be able to prepare the test population. A dominant stress factor may be sufficient for preparing the injured population; however exposure of the population to as many factors as possible to mimic the real world environment is advised. Again, it is best to expose test organisms to the stress agents of the test matrix, for example, in-process samples that have osmolar and acidic characteristics can be used to generate that injury in spiked microbes. if this is not possible, then the sample matrix should mimic the normal environment as much as possible usually by modeling the inherent stress factors artificially.
Following selection of the specific stress factors, test organisms, and method, the level of exposure required to cause injury in a target microbial population must be experimentally defined. This will consist of determining an appropriate intensity and time of exposure to the stress agent to induce injury. The degree of injury can be determined through comparing the level of recovery of an injured population on selective versus non-selective media, or measuring the overall degree of kill of the stressed population. in the first instance, use of a selective media will counter-select injured over non-injured CFUs. it is also acceptable to use the principle that a population is stressed when at least 90% of the cells have been rendered non-viable by the treatment in question [7]. This would be established by comparing a number of surviving CFU of the treated population versus the untreated control culture. once the appropriate method has been determined, a stressed population can be prepared. Using this population, the effectiveness of a new application can be examined as to whether it can detect and enumerate injured cells compared to unstressed populations.
Effects of Stress on Growth Dynamics of a Microbial Population
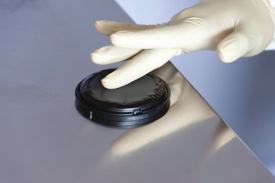
The efficacy of this technique and effect of stress can be tracked on the Growth direct™ System. The Growth direct System technology detects microscopic colonies well before they become visible to the eye by imaging their intrinsic cellular autofluorescence [8]. The detection technology of the Growth direct System exploits the fact that all microbial cells emit yellow-green fluorescence when illuminated by blue light due to the presence of fluorescent biomolecules inclusive of (ribo)flavins and flavoproteins [9-11]. like ATp, these molecules are ubiquitous in living systems in all the kingdoms of life including microorganisms. The automated digital imaging of autofluorescent microbial colonies significantly reduces the detection time of a growing colony over that of the compendial method by approximately 50% on average by interrogating regular images of the membrane surface, and delineating growing colonies from inanimate debris by the change in fluorescent signal. The Growth direct System is particularly suited for examining injured populations as this regular image interval will detect colonies shortly after the microbe begins to grow.
An example of the effect of stress/injury on a microbial population is presented in Figure 1 that compares the detection of heat-injured B. subtilis with an uninjured population on the Growth direct system. The B. subtilis population was subject to heat treatment sufficient to obtain an appropriate level of injury as shown by a 90% kill versus the untreated starting population. Both the stressed and unstressed samples were analyzed on the Growth direct System, and the cumulative detection of growing colonies tracked over time as shown. As discussed above, injured cells (or spores as well) exhibit an extended lag due to the time taken to recover from injury. The lag will vary dependent on the degree of injury sustained by each colony forming unit. The plot of the % detection of the stressed and unstressed B. subtilis delineates the effects of a lag experienced by the cells in the stressed population. individual cells only will grow once cell damage is repaired, thus an extended detection curve is indicative of cells requiring different time periods to recover. in contrast, the sharply vertical detection curve of the unstressed control is consistent with cells that need no recovery time and therefore begin growing almost immediately.
Figure 1. Recovery dynamics of heat-stressed Bacillus subtilis during analysis on Growth Direct SystemThe Potential Practical Impact of Stressed Populations is Closer than One Thinks
Effects of stressed populations may also affect testing results even when using microbial populations that are commonly used. For example, USp/Ep specified test organisms are regularly used in new application validation testing. in many cases, such testing utilizes commercial preparations of these microbial strains. Such preparations vary in their manufacturing process, and thus may have been injured to different degrees during processing. As described, the level of injury will affect the time required for the colonies to grow to a detectable size, whether by standard or rapid method, and thus affect the time-to-result of an assay. This possibility was examined by comparing the time-to result of the five USp test organisms obtained from three commercial vendors as lyophilized preparations as well as an in-house overnight growth preparation (Table 2). The four bacteria were tested by growth on TSA incubated for 48 hours at 32°C, while A. brasiliensis was incubated at 22°C for 96 hours. presumably, the time-to-result values should not vary significantly between the different samples tested. A longer timeto result would be indicative of preparations that are more stressed. The time-to result values in Table 2 show the significant variation in performance present between the different vendor preparations of commonly used test strains. This variation presumably occurs due to effects of the manufacturing process that stress the test populations to different degrees.
Table 2. Time to result detection (hrs) for the five USP organisms on the Growth Direct System shows vendor to vendor variation
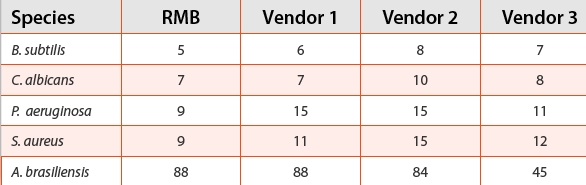
Conclusion
it has been recognized that the validation of new microbial test methods should utilize challenge samples that at least model in situ microbial populations either by using such populations themselves, or by using model populations prepared in the laboratory. This validation strategy better represents the organisms in the environment that exist in a constant state of stress, and that often have experienced some form of cell injury by the time they are captured for culture by the microbiologist. The example presented here is just one example that demonstrates the difference in the growth and recovery that can exist between a non-stressed and an injured population of organisms. possible stress effects can even have an impact on testing results even when commercially prepared microbial populations are used. This effect is less likely to be seen for the traditional method, but has more significance for the faster RMMs. in either case, the specifications of a “validated” new application may be derived from results that were obtained from unsuitable test populations. Thus, contaminating bioburden perhaps would not be detected within the time-frame of the test application as validated with serious consequences arising as a result.
Author Biography
Andrew Sage, Ph.D., has more than fifteen years of experience with rapid microbiology. Since 2009, Dr. Sage has served as the Manager of Microbiology Applications focused on the implementation of new methods for the Growth Direct System. He joined Rapid Micro Biosystems in 2004 as principal scientist where he has worked on a number of projects associated with the development and commercialization of the Growth Direct™ system. Previously Dr. Sage worked at Millipore Corporation as a senior scientist in their Bioprocess Division where he focused on ATP-bioluminescence and peptide nucleic acid probe detection technologies. Andrew earned a B.S. in biology from The College of William and Mary, and a doctorate in microbiology from the University of Massachusetts-Amherst.
References
- Validation of Growth-Based Rapid Microbiological Methods for Sterility testing of Cellular and Gene Therapy Products, 2008 Draft Guidance. Food and Drug Administration
- Gray, J.C.; Staerk, A.; Berchtold, M.; Hecker, W.; Neuhaus, G.; and Wirth, A. 2010 Growthpromoting properties of different solid nutrient media evaluated with stressed and unstressed micro-organism: Prestudy for the evaluation of a rapid sterility test. PDA J. Pharm. Sci. Technol. 64 249-263
- Wesche, A.M.; Gurtler, J. B.; Marks, B. P.; Ryser, E.T.; Stresss, Sublethal injury, resuscitation, and virulence of bacterial foodborne pathogens 2009 J. Food Prot. 72 121-138
- Stortz, G., Henge, R. (Ed.) Bacterial Stress Response, 2nd ed. 2011 ASM Press Washington DC
- Sandle, T. 2011A Review of cleanroom microflora: types, trends, and patterns PDA J. Pharm. Sci. Technol. 64 249-263
- Dupont, C.; Augustin, J. 2009 Influence of stress on single-cell lag time and growth probability for Listeria monocytogenes in half fraser broth App. Environ Microbiol. 75 3069-3076
- Stephens, P. J.; Joynson, J. A.; Davies, K. W.; Holbrook, R.; Lappin-Scott, H. M.; Humphrey, T. J. 1997. The use of an automated growth analyser to measure recovery times of single heatinjured Salmonella cellsJ. App. Microbiol. 83 445-455
- London, R: Schwedock, J.; Sage, A., Valley. H; Meadows, J.; Waddington, M.; Straus, D. 2010 An Automated System for Rapid Non-Destructive Enumeration of Growing Microbes. PLoS ONE 5(1): e8609. doi:10.1371/journal.pone.0008609
- Aubin, J. E. 1979 Autofluorescence of viable cultured mammalian cells. The J. Histochem. Cytochem. 27 36-43
- Dalterio, R; Nelson, W.; Britt, D.; Sperry, J.; Tanguay, T.; Suib., S. 1987 The Steady-State and Decay Characteristics of Primary Fluorescence From Live Bacteria. Appl. Spect., 41 234-240
- Estes, C.; Duncan, A.; Wade, B.; Lloyd, C.; Ellis, W.; Powers.; P. 2003. Reagentless detection of microorganisms by intrinsic fluorescence. Biosens. Bioelectron. 18: 511-9