- Abbvie Bioresearch Center
In an increasingly competitive landscape for the launch of new biopharmaceutical products, there are significant efforts within the industry to reduce the time-to-market, in an attempt to secure market share and maximize the duration of market exclusivity. In addition, the FDA has introduced the breakthrough therapy designation to expedite program timelines for treatments that target serious diseases. Since the first breakthrough therapy approval in 2012, an additional 144 programs have received this designation through the end of the 2016 fiscal year.1 The increased emphasis on shortened program timelines can result in Chemistry, Manufacturing and Controls (CMC) activities moving to the critical path for enabling product launch. Among the key CMC activities that could be rate-limiting is manufacturing process characterization. This article reviews strategies to increase the rate and quality of process characterization, with an emphasis on the manufacturing of antibodies and related protein therapeutics.
Process characterization plays a key role in establishing the manufacturing process control strategy by defining the unit operations and operating ranges that allow the process to generate product that meets the Quality Target Product Profile (QTPP). The QTPP defines the acceptable values for a product’s Critical Quality Attributes (CQAs), which are properties that ensure safety and efficacy for patients.2 Like process development, process characterization is performed using representative laboratory scale down models (SDMs) and typically involves multiple activities to ensure the robustness of the process, including range finding studies, process mapping, impurity spiking/ clearance studies, cleaning/storage studies, resin/membrane aging studies, virus clearance validation studies, and leachable/extractable analyses. Range finding studies serve as the centerpiece to process characterization, in that they define the Proven Acceptable Ranges (PARs) of the process operating parameters. Range finding studies are typically comprised of univariate or multivariate experiments in which process parameters are tested above and below their target values, either individually or in combination with one another, resulting in a comprehensive and predictive data set within the ranges evaluated.
Acceleration Through Process Design
Enabling accelerated process characterization can begin early in the project lifecycle during Phase I process development. In this scenario, the design of clinical manufacturing processes should ensure that the QTPP and process robustness are suitable for potential commercial launch. This avoids any major re-work of the process during later phases of the program. In addition, development of commercial ready cell lines should provide the desired level of productivity and meet the expectations of regulatory agencies for cell line clonality and stability. This will avoid the need to change cell lines at a later time, which presents risks associated with product comparability. In addition, the establishment of robust platform analytical methods is valuable in avoiding the need to modify analytical assays later in the program, as this may slow progress if there are challenges related to assay output variability. Overall, this commercial-ready development scheme is most suitable for organizations that are experienced in process development and product launch, and have considerable process knowledge and expertise to support the process design. For less experience organizations, this strategy may require additional upfront effort and resources which may be an excessive investment for early-phase programs.
A key resource in the early-phase development of commercial-ready processes is the ability to leverage platform process experience for upstream and downstream manufacturing. Platform manufacturing processes are defined by a standardized set of unit operations and operating parameters that deliver consistent and robust performance across comparable programs. These are particularly applicable for molecules such as monoclonal antibodies, which have a relatively high level of similarity in their biophysical characteristics. Platform processes allow for more extensive early-phase process understanding, which improves the ability to set optimal process operating conditions early on, mitigating the risk of significant latestage process changes. Avoiding late-stage changes accelerates process characterization timelines by minimizing the time spent re-developing process unit operations, while allowing prior process knowledge to be leveraged in the design of characterization experiments. Prior knowledge can also streamline the time invested in process characterization-related activities, such as risk assessments, statistical study design, study execution, and data analyses. Avoiding major late-phase process changes also maintains the relevance of existing manufacturing history data, which can be valuable when planning Process Performance Qualification (PPQ) activities and assessing which operations within the manufacturing process require higher levels of control. In addition, minimizing latephase changes ensures that existing stability data for early-phase drug substance remains representative for the late-phase drug substance as well.
Another strategy to accelerate process characterization through process design is by utilizing single-use materials. For example, substituting chromatography resins and UF/DF membranes with single-use membrane technologies can eliminate the need to qualify the lifetime performance of the resins/membranes. This can save weeks of resin/membrane aging and cleaning/storage studies, as well as significantly reducing the quantities of process intermediates needed to perform the studies.
Acceleration Through Experimental Design
The traditional timing for process characterization activities has been to perform them approximately between the timeframes of Phase II and Phase III clinical trials. This timing helps to avoid investing extensive resources too early before clinical efficacy of a molecule has been established. However, for fast-moving clinical programs, this timing increases the risk that process characterization activities may move to the critical path for commercial launch. To avoid potential delays, it may be appropriate to move process characterization activities further upstream in the process lifecycle. This can be challenging, as process characterization can require significant resources in terms of headcount, financial cost, and supply of process intermediates with which to perform the studies. These challenges may be addressed through strategic experimental design and execution.
One strategy is the use of automated high-throughput technologies, such as miniaturized bioreactor models for cell culture and small-volume liquid handling robots for chromatography.3 These technologies allow the ability to perform large numbers of experiments in parallel, while greatly reducing the investment of time and materials. Miniature high-throughput screening can be highly effective in identifying which operating parameters significantly impact product CQAs, while eliminating non-significant parameters from future studies. In some cases miniaturized scale-down models may have an off-set in process performance and product quality attributes as compared to more traditional laboratory SDMs, so traditional SDMs may be more appropriate for defining the PARs of statistically significant parameters.4 Still, the use of miniaturized high-throughput screening can greatly improve the efficiency of range finding studies by eliminating the parameters that do not significantly impact CQAs.
Another strategy to reduce the upfront investment required for early process characterization activities is the use of efficient statistical screening designs. These study designs are implemented in order to study the effect of multiple process parameters in combination with one another, across a wide range of operating parameters. They decrease the number of multivariate runs required, while allowing the ability to resolve univariate effects and multivariate interaction effects on CQAs. A recent advancement in statistical screening designs is the introduction of Definitive Screening Design (DSD). DSD can be more efficient than traditional fractional factorial and Plackett-Burman screening designs, and offers the ability to resolve quadratic effects, which the traditional screening designs are unable to accomplish.5 Identifying quadratic effects during screening studies may provide the ability to avoid more extensive follow-up statistical studies, such as response surface designs.
These experimental design strategies could potentially remove months from process characterization timelines, or allow more in-depth analysis of process parameter effects within similar timelines to traditional experimental approaches for process characterization.
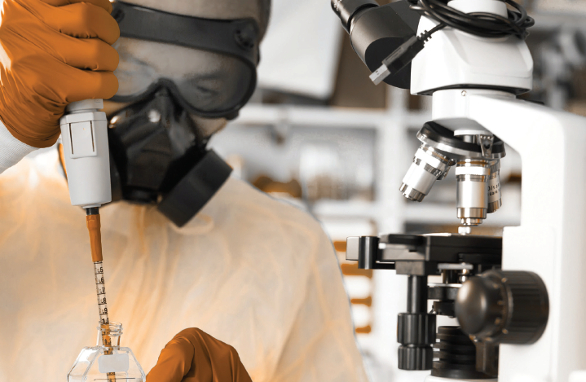
Acceleration Through Project Timelines
A further approach for accelerating project timelines is in the prioritization of process characterization activities within the project timeline. If the goal is to complete process characterization rapidly to enable PPQ runs, it may be acceptable to divide process characterization into two or more tiers, with early tiers enabling PPQ and later tiers performed in-parallel with or following the PPQ runs. For example, early tier range-finding studies may be acceptable to define PARs and identify parameters to be monitored during validation. Later tier studies could then be used for further evaluation of some high impact parameters, if necessary, as well as other process characterization activities such as resin aging studies and impurity spiking/clearance studies. A caveat for this approach is that additional studies and documentation would be needed if post-PPQ studies were to result in unexpected findings. Hence, this strategy is most amenable to a situation in which a robust platform process is in place and the reliability of the process can be predicted with a high level of confidence. This strategy can provide a program timeline compression of up to 6-12 months over the traditional approach of completing process characterization prior to proceeding to PPQ.
Strategic Implementation for Acceleration
The strategies described offer a number of opportunities to accelerate process characterization and potentially prevent CMC activities from moving to the critical path for product launch. They could be implemented independently or in combination with one another, based on the target timelines of the program and the experience of the organization. Successful acceleration of process characterization activities is feasible and should be based on an integrated strategy among the process development, analytical, manufacturing, CMC, and regulatory representatives of the program.
References
- “Frequently Asked Questions: Breakthrough Therapies.” Regulatory Information U.S. Food and Drug Administration, 06 June 2016. Website: http://www.fda.gov/ RegulatoryInformation/Legislation/SignificantAmendmentstotheFDCAct/FDASIA/ ucm341027.htm
- ICH Q8(R2): Pharmaceutical Development, Step 4. International Conference on Harmonisation of Technical Requirements for Registration of Pharmaceuticals for Human Use: Geneva, Switzerland, 1 Aug. 2009.
- Jones, S., Ransohoff, T., Castillo, F., Riske,F., Levine, H. (2015). “High Throughput Process Development Approaches for Biopharmaceuticals”. American Pharmaceutical Review Website: https://www.americanpharmaceuticalreview.com/Featured-Articles/172881- High-Throughput-Process-Development-Approaches-for-Biopharmaceuticals/
- Petroff, M., Bao, H., Welsh, J., van Beuningen – de Vaan, M., Pollard, J., Roush, D., Kandula, S., Machielsen, P., Tugcu, N., Linden, T. (2016) “High throughput chromatography strategies for potential use in the formal process characterization of a mononoclonal antibody.” Biotechnology and Bioengineering 113(6); 1273-83.
- Jones, B. and Nachtsheim, C. (2011) “A class of three-level designs for definitive screening in the presence of second-order effects.” Journal of Quality Technology 43.1.
About the Author:
Michael Clark has been a Senior Scientist at the Abbvie Bioresearch Center in Worcester, MA since 2007. During that time he has supported the development and characterization of several antibody and antibody-drug conjugate processes, including authoring related sections in regulatory findings.