Traditional bioprocesses of mammalian cell cultures are operated in batch- or fed-batch mode. To increase space-time-yields, reduce cost of goods, accelerate time-to-market and enhance flexibility at reduced lab-footprint, perfusion processes are envisaged for various bioprocess intensification tasks. The goal of dynamic perfusion is to achieve high viable cell densities (VCD) by maintaining high specific growth rates over prolonged time-periods. It is usually operated at high perfusion rates to generate the required biomass for the establishment of high-density cryopreserved cell banks, or as inoculum in the N-1 stage for larger production bioreactors operated as (intensified) fed-batch or concentrated fed-batch cultures. Dynamic perfusion operation can be switched to a continuous, steady-state perfusion culture at low perfusion rates and constantly high VCD over several weeks or even months for efficient product generation at high quality. Depending on the specific goal of perfusion operation a suitable medium should support maximum growth or productivity.
The optimal combination of perfusion media and process conditions extends the viable cell concentration and the integral of viable cell density (IVCD, also: cell days) far beyond what can be reached with batch-based cultivation (Figure 1A). The obtained product yields are markedly higher by increased IVCD values when cell-specific productivity (qP) can be maintained (Figure 1B). One of the biggest challenges is the cumulative consumption of nutrients and production of toxic waste products, exemplified here for glucose and lactate (Figure 1C and D). The slope of an IVCD-graph allows deeper insight into the cellular performance and expresses common cell culture parameters including cell-specific growth (μ), -productivity (qP), -glucose consumption (qGluc) and –lactate production (qLac).
The nutrient level in perfusion cultures can be controlled at two levels. First, by the medium composition and, second, by the feed flow entering the bioreactor comparable to fed-batch optimization. One profound difference is that in perfusion processes the product as well as toxic by-products are removed from the culture by varying the continuous flow of spent medium, while in fed-batch processes toxic products are maintained inside the culture vessel and the product is additionally exposed to enzymes released from dying cells. Consequently, balanced perfusion flow rates have to be developed for a given cell-line and the corresponding medium to deliver enough nutrients and effectively remove toxic waste products.
The Concept of Low Cell-Specific Perfusion Rates (CSPR) as a Key Marker for High-Performing Perfusion Media
Optimized perfusion media support a healthy cellular physiology at minimal perfusion rates. A typical design goal for new media is to reach lowest cell-specific perfusion rates (CSPR) in order to decrease overall media consumption, increase harvest titer, reduce storage-tank size and lab footprint. In essence, the CSPR describes the perfused medium volume per cell per day. Lowest CSPRs of 20 pL/cell/day were already described in literature. A spherical CHO cell with an average cell diameter of 13 μm is defined by 1 pL of cell mass, meaning that a 20-fold excess of the cell volume passes the reactor at a CSPR of 20 pL/cell/day. Assuming further that fast growing cells have a specific glucose consumption rate of up to 400 pg/cell/day, our perfusion medium has to supply 400 pg glucose in 20 pL medium volume translating into a glucose concentration of 20 g/L. If we increase the perfusion rate to a CSPR of 40 pL/cell/day our medium has to contain only 10 g/L to support the cellular glucose demand, whereas if we want to push the CSPR down to 10 pL/cell/day we have to supply 40 g/L of glucose. Such calculations are also valid for toxic waste compounds. We observed that rapidly growing cells produce up to 50 pg/cell/day of lactate. This results in a fi nal lactate concentration of 2.5 g/L at a perfusion rate of 20 pL/cell/day. If we reduce the CSPR to 10 pL/c/d this translates into 5 g/L of lactate or decreases to 1.3 g/L at a higher perfusion rate of 40 pL/cell/day. Highly productive recombinant cell lines may produce up to 20 pg/cell/day of recombinant protein. At a CSPR of 10, 20 and 40 pL/cell/day this accumulates into titers of 2, 1 and 0.5 g/L respectively.
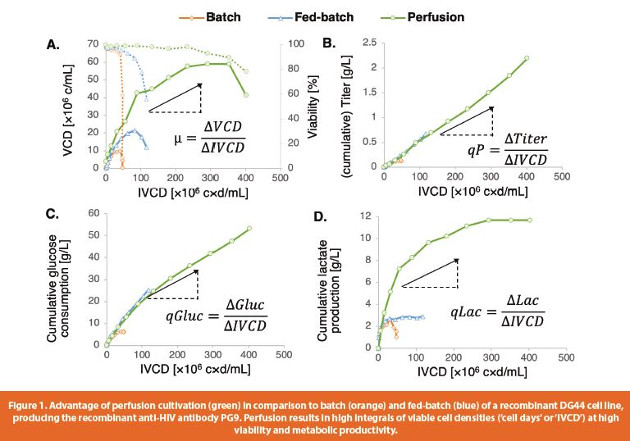
As we push the boundaries towards lowest CSPRs in perfusion cultures, we have to apply highly concentrated media and carefully consider lower washout and critical concentrations of toxic metabolites. Furthermore, the residence time of the protein products is increased at lower perfusion rates. For stable products such as antibodies, lower perfusion rates are preferable and result in much higher product titers in the harvest flow and makes perfusion an attractive concept for bioprocess intensifi cation using single-use equipment in a compact and portable format. The development of highly concentrated perfusion media for operation at low CSPRs also allows us to apply the chemostat mode. Running a chemostat demands to support low dilution rates due to low specific growth rates of mammalian cells compared to bacteria.
Leveraging Existing Platform Media for Design of Novel Perfusion Media
Comparable to traditional batch-based processes, the cell culture medium profoundly determines the perfusion culture performance, the final product yield and the product quality. Cell culture media are designed for improved cellular physiology (growth and productivity), proper physicochemical properties (viscosity, reduced foaming, proper solubility of oxygen, pH, buffer capacity, etc.) and stability (storage, shelf-life, degradation). So-called platform media are developed for multiple steps in the upstream processing, including thawing, routine cultivation, biomass expansion, production and cryopreservation. Nowadays, a broad spectrum of basal media and diff erent feeding supplements are available to obtain a balanced set of carbohydrates, amino acids, lipids, vitamins, organic acids and trace elements.
Subscribe to our e-Newsletters
Stay up to date with the latest news, articles, and events. Plus, get special offers
from American Pharmaceutical Review – all delivered right to your inbox! Sign up now!
In the past, such off-the-shelf media were usually designed specifically for traditional fed-batch applications and a lot of knowledge about how media components influence cell cultures has accumulated over the last decades. It is now our task to combine this broad body of knowledge and leverage it for new bioprocesses. One strategy is to use existing platform media and combine it with certain supplements for perfusion.1,2 In fed-batch processes it is necessary to supply a balanced set of nutrients by accurately timed addition of the highly concentrated feed solutions to keep culture osmolality low. Nutrients are maintained by a daily bolus- or continuous feed, but toxic by-products accumulate and stay within the culture. The optimization is achieved by a.) the composition of the highly concentrated feed solution and b.) defining the best feeding regimen. In contrast, a perfusion medium delivers nutrients by a continuous medium flow and in parallel removes toxic waste products. Therefore, the development of perfusion processes involves optimization of a.) composition of the perfusion medium and b.) defining the best perfusion rate. Despite the differences in medium composition and process control, beneficial properties of a platform medium can be transferred across multiple modes of operation and fine-tuned for continuous perfusion or chemostat cultivation.
Supporting Techniques for Perfusion Media Development
Although various complex media and feed solutions are available and can be used off-the-shelf as a starting point for specifically designing a novel perfusion medium, many variables have to be screened to find an optimum concerning nutrient concentration, osmolality, pH, buffer capacity, oxygen/CO2 solubility and the perfusion rate at high cell densities. For successfully fulfilling this complex task, multifactorial approaches and tools are needed to find the global optimum.
Traditional methods for cell culture medium design involve rational approaches such as spent medium analyses to identify limiting or toxic compound-concentrations, as well as empirical approaches such as media blending to combine the benefits of multiple individual media solutions. Chromatographic analysis by HPLC, GC, LC-MS and GC-MS or NMR- and RAMAN spectroscopy can help to identify unnecessarily high nutrient concentrations in the perfusion harvest and to detect a nutrient steady-state at physiological conditions.
Design of Experiment (DoE) in combination with response-surface modelling have been proven especially advantageous compared to traditional one-factor-at-a-time (OFAT) approaches to identify optimal ‘factors’ (i.e. substances), their ‘levels’ (i.e. concentrations) and ‘factor interactions’. Furthermore, DoE reduces experimental costs and extracts maximum information from a limited number of experiments when many different factors and levels have to be screened and optimized.
Investigation of a large number of different culture conditions requires the application of many parallel cultures that can be operated at small volumes in high-throughput with a minimum of required resources. Robust and reproducible small-scale models were typically developed for batch- and fed-batch applications but are now also required for perfusion process development to simulate the performance of larger bioreactor cultures and to predict key parameters including cellular productivity, protein quality and critical process parameters such as minimal CSPR or optimal daily cell bleed volumes. In contrast to batch/fed-batch models, continuous media addition and harvest removal must be made possible with proper techniques for effective cell retention or controlled cell bleed. Because of the higher complexity and lack of suitable cell-retention devices at small scale, only a limited number of small-scale concepts and miniaturized bioreactors are available. Small-scale models are preferably operated at a working volume of few milliliters to reduce overall media consumption. This means that very low media flow rates must be applied when operated continuously. Flow rates as low as 7 μL per minute must be supported for a working volume of 10 mL at a daily perfusion rate of 1 vvd. For a true perfusion with a continuous media flow this requires the availability of proper microfluidics systems. An alternative is to operate the liquid transfer in defined intervals. An average perfusion volume of 10 mL per day, for example, may be realized by using small peristaltic pumps at 0.5 mL/min. for 5 minutes every six hours, or for 20 minutes once a day. However, deviating from a truly continuous perfusion operation in such semi-continuous cultures results in fluctuating peak titer and metabolite profiles that may profoundly impact cellular behavior and final product quality and has to be evaluated carefully.
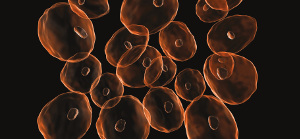
Semi-continuous perfusion cultivation is defined by a daily media exchange using centrifugation for separation of the cell culture supernatant and simulates the perfusion mode in simple shaking tubes or -flasks which are readily available in any cell culture lab. This cost-effective cultivation model can be operated in high-throughput by a single operator and provides an attractive small-scale alternative for perfusion processes. However, standard shaking tubes are not equipped with sensors and controllers for pH and dissolved oxygen (DO). As a consequence, recent efforts are made to develop more complex miniaturized bioreactors operated at truly continuous perfusion at very small working volumes (50-200 mL) with proper DO and pH control.
Challenges and Future Directions
Once a suitable perfusion medium is defined by rational or empirical approaches using proper small-scale models, the final medium has to meet several attributes of quality, stability and manufacturability. Powder formulations of media are preferred for handling purposes to extend the shelf-life, minimize the storage volume and facilitate shipping to different locations. Proper protocols for liquid reconstitution of all components have to be developed and may include pelleting of the medium powder to speed up hydration time at large scale. Large media volumes, required for prolonged (steadystate) perfusion runs are provided by dilution of media concentrates on-site to reduce storage- and lab space. Proper methods should be implemented for detection, monitoring and control of medium degradation caused by thermal- and light energy and may include spectroscopic (RAMAN) methods combined with chemometric tools.
Highly enriched perfusion media supporting very low CSPRs down to 20-40 pL/cell/day were already developed but this creates two challenges: First, for small-scale models very low flow rates are required by using microfluidics systems in continuous operation. Alternatively, conventional peristaltic pumps are operated in defined time-intervals. Second, toxic by-products are accumulating at low perfusion rates. Therefore, it is mandatory to identify all toxic cell metabolites and to understand their metabolic network to finally control their biosynthesis by cellular (metabolic) engineering, media design or process control.3–7
Recent approaches for media design also aim to include methods of systems biology to understand the impact of culture environment on cellular physiology in more detail. Various -omics techniques are available that support traditional media development. We used transcriptomics to investigate the cellular impact of feed supplements on cell lines with high-productivity.8 Also, metabolic flux analysis or characterization of the cellular proteome are highly suitable concepts to understand and modify the cellular physiology by media design in a product- and cell-specific manner. Novel approaches aim to design culture media in-silico by mechanistic modeling using stoichiometric or kinetic models. The fact, that production titers have increased significantly will in the near future challenge media development for optimizing the product quality. In case of antibodies charge-, size- and glyco-isoform distribution are defined as such critical quality attributes.
References
- Lin H, Leighty RW, Godfrey S, Wang SB. Principles and approach to developing mammalian cell culture media for high cell density perfusion process leveraging established fed-batch media. Biotechnology Progress. 2017;33(4):891-901. doi:10.1002/btpr.2472
- Mayrhofer P, Reinhart D, Castan A, Kunert R. Rapid development of clone-specific, high-performing perfusion media from established feed supplements. Biotechnology Progress. n/a(n/a):e2933. doi:10.1002/btpr.2933
- Mulukutla BC, Kale J, Kalomeris T, Jacobs M, Hiller GW. Identification and control of novel growth inhibitors in fed‐batch cultures of Chinese hamster ovary cells. Biotechnology and Bioengineering. 2017;114(8):1779-1790. doi:10.1002/bit.26313
- Toussaint C, Henry O, Durocher Y. Metabolic engineering of CHO cells to alter lactate metabolism during fed-batch cultures. Journal of Biotechnology. 2016;217:122-131. doi:10.1016/j.jbiotec.2015.11.010
- Mulukutla BC, Mitchell J, Geoffroy P, et al. Metabolic engineering of Chinese hamster ovary cells towards reduced biosynthesis and accumulation of novel growth inhibitors in fedbatch cultures. Metabolic Engineering. 2019;54:54-68. doi:10.1016/j.ymben.2019.03.001
- Gagnon M, Hiller G, Luan Y-T, Kittredge A, DeFelice J, Drapeau D. High-end pH-controlled delivery of glucose effectively suppresses lactate accumulation in CHO fed-batch cultures. Biotechnol Bioeng. 2011;108(6):1328-1337. doi:10.1002/bit.23072
- Ma N, Ellet J, Okediadi C, Hermes P, McCormick E, Casnocha S. A single nutrient feed supports both chemically defined NS0 and CHO fed-batch processes: Improved productivity and lactate metabolism. Biotechnol Prog. 2009;25(5):1353-1363. doi:10.1002/btpr.238
- Reinhart D, Damjanovic L, Castan A, Ernst W, Kunert R. Differential gene expression of a feed-spiked super-producing CHO cell line. Journal of Biotechnology. 2018;285:23-37. doi:10.1016/j.jbiotec.2018.08.013
About the Authors
Patrick Mayrhofer is Senior Scientist at the Department of Biotechnology (DBT) at the BOKU University Vienna, where he is responsible for the bioprocess development at the research group ‘Animal Cell Factory Design’ of Prof. Renate Kunert. He exploits various cell culture technologies in batch-/fed-batchand continuous operation together with media optimization to improve extrinsic- and intrinsic culture conditions for maximum yield and quality of monoclonal antibodies and difficult-to-express proteins.
Renate Kunert is full professor and chair of the Institute of Animal Cell Technology and Systems Biology at the Department of Biotechnology (DBT) at the BOKU University Vienna. The focus of her research group is the design of difficult-to-express proteins (e.g. CD19) and different antibody isotypes (IgG, IgM, IgA), to establish and select high-producing cell lines and to optimize upstream bioprocesses for enhanced control of cellular physiology, improved yield and quality attributes.